Inhibícia 12/15 lipoxygenázy kurkumínom a extraktom z Curcuma longa L.
Autoři:
Lýdia Bezáková; Daniela Košťálová; Marek Obložinský; Peter Hoffman; Mária Pekárová; Renáta Kollárová; Ivana Holková; Silvia Mošovská; Ernest Šturdík
Působiště autorů:
Comenius University, Faculty of Pharmacy
Vyšlo v časopise:
Čes. slov. Farm., 2014; 63, 26-31
Kategorie:
Původní práce
Souhrn
Kurkumín (diferuloylmetan) je oranžovo-žlto sfarbená obsahová látka – sekundárny metabolit z rizomy (Curcuma longa L.), korenia (turmeric) často prítomného v prášku curry. Je to jeden z hlavných kurkuminoidov turmeric Curcuma longa L. Po stáročia bol kurkumín používaný v niektorých liečivých preparátoch, alebo ako korigens farby v potravinách. Ukázalo sa, že viaceré enzýmy, ktoré sú úzko spojené so zápalovým a nádorovým procesom, sú modulované kurkumínom. V tejto práci sú sumarizované výsledky inhibičného efektu kurkumínu a etanolického extraktu z Curcuma longa L. na aktivitu lipoxygenázy (LOX) z cytozolovej frakcie pľúc potkana. Stanovenie polohovej špecifity dioxygenácie kyseliny arachidónovej RP- and SP-HPLC metódou ukázalo, že v purifikovanom enzýmovom preparáte z cytozolovej frakcie pľúc potkana je prítomná špecifická forma lipoxygenázy preukazujúca 12/15-LOX duálnu špecifitu (s prevažujúcou 15-LOX aktivitou). Inhibičná aktivita kurkumínu a extraktu z Curcuma longa na aktivitu lipoxygenázy z cytozolovej frakcie pľúc potkana bola vyjadrená v percentách inhibície a hodnotách IC50. Vyjadrením kinetiky enzýmovej reakcie podľa Lineweaver-Burk za prítomnosti inhibítora sa zistilo, že kurkumín je kompetitívny inhibítor 12/15- LOX z cytozolovej frakcie pľúc potkana.
Keywords:
Curcuma longa L., kurkumín, turmeric extrakt, kyselina linolová, inhibícia lipoxygenázy
Zdroje
1. Jayaprakasha G. K., Jagan L., Rao M., Sakariah K. K. Chemistry and biological activities of C. longa. Trends in Food Science Technology 2005; 16, 533–548.
2. Gupta S. C., Prasad S., Kim J. H., Patchva S., Webb L., Priyadarsini I. K., Aggarwal B. B. Multitargeting by curcumin as revealed by molecular interaction studies. Nat. Prod. Rep. 2011; 28, 1937–1953.
3. Ak T., Gúlcin I. Antioxidant and radical scavenging properties of curcumin. Chemico-Biological Interaction 2008; 174, 27–37.
4. Basnet P., Skalko-Basnet N. Curcumin: An anti-inflammatory molecule from a curry spice on the path to cancer treatment. Molecules 2011; 16, 4567–4598.
5. Shehzad A., Khan S., Shehzad D., Lee Y. S. Curcumin therapeutic promises and bioavailability in colorectal cancer. Drug Today 2010; 47(7), 523–532.
6. Caroll R. E., Bena R. V., Turgeon D. K., Varee S., et al. Phase II clinical trial of curcumin for the prevention of colorectal neoplasia. Cancer Prev. Res. 2011; 4(3), 354–364.
7. Shureiqi I., Baron J. A. Curcumin Chemoprevention: The long road to clinical translation. Cancer Prev. Res. 2011; 4(3), 296–298.
8. Ammon H. P., Safayhi H., Mack T., Sabieraj J. Mechanism of anti-inflammatory actions of curcumine and boswellic acids. J Ethnopharmacol. 1993; 38(2–3): 113–119.
9. Kohll K., All J., Ansari J., Raheman Z. Curcumin: A natural antiinflammatory agent. Indian J. Pharmacol. 2005; 37(3), 141–147.
10. Jankun L., Aleem A. M., Malgorzewicz S., Szkudlarek M., Zavodszky M. I., Devitt D. L., Feig M., Selman S. H., Skrzypczak-Jankun E. Synthetic curcuminoids modulate the arachidonic acid metabolism of human platelet 12-lipoxygenase and reduce sprout formation of human endothelial cells. Mol. Cancer Ther. 2006; 5(5), 1371–1382.
11. Wu J., Xiang Xia H. H., Ping Tu S., Fan D. M., Lin M. Ch., Kung H. F., Lam S. K., Wong B. Ch. 15-lipoxygenase-1 mediates cyclooxygenase-2 inhibitor-induced apoptosis in gastric cancer. Carcinogenesis. 2003; 24(2), 243–247.
12. Segraves S., Walther M , Ivanov I., et al. Sequence determinants for the reaction specificity of murine (12R)-lipoxygenase: targeted substrate modification and site-directed mutagenesis. J. Biol. Chem. 2005; 280, 36633–36641.
13. Werz O., Steinhilber D. Therapeutic options for 5-lipoxygenase inhibitors. Pharmacol. Therapeut. 2006; 112: 701–718.
14. Montuschi P., Sala A., Dahlén S. E., Folco G. Pharmacological modulation of the leukotriene pathway in allergic airway disease. Drug Discov. Today 2007; 12, 404–412.
15. Wen Y., Gu J., Vandenhoff G. E., Liu X, Nadler J. L. The role of 12/15‑lipoxygenase in the expression of MCP-1 in mouse macrophages. Am. J. Physiol. Heart Circ. Physiol. 2008; 294, H1933–H1938.
16. Chakrabarti S. K, Cole B. K, Wen Y., Keller S. R., Nadler J. L. 12/15‑lipoxygenase products induce inflammation and impair insulin signaling in 3T3‑L1 adipocytes. Obesity 2009; 17, 1657–1663.
17. Yuan H., Lanting L., Xu Z. G., Li S. L., Swiderski P., Putta S., Jonnalagadda M., Kato M., Natarajan R. Effects of cholesterol-tagged small interfering RNAs targeting 12/15‑lipoxygenase on parameters of diabetic nephropathy in a mouse model of type 1 diabetes. Am. J. Physiol‑Renal 2008; 295, F605–F617.
18. Martínez-Clemente M., Ferré N., Titos E., Horrilo R., González-Périz A., Morán-Salvador E., López-Vicario C., Miquel R., Arroyo V., Funk C. D., Claria J. Disruption of the 12/15‑lipoxygenase gene (Alox15) protects hyperlipidemic mice from nonalcoholic fatty liver disease. Hepatology 2010; 52, 1980–1991.
19. Krönke G., Katzenbeisser J., Uderhardt S., Zaiss M. M., Scholtysek C., Schabbauer G., Zarbock A., Koenders M. I., Axmann R., Baencler H. W, van den Berg W., Voll R. E., Kuhn H., Joosten L. A., Schett G. 12/15‑lipoxygenase counteracts inflammation and tissue damage in arthritis. J. Immunol. 2009; 183, 3383–3389.
20. Rossaint J., Nadler J. L., Ley K., Zarbock A. Eliminating or blocking 12/15-lipoxygenase reduces neutrophil recruitment in mouse models of acute lung injury. Crit. Care 2012; 16, R166.
21. Kulkarni A. P., Cai Y., Richards I. S. Rat pulmonary lipoxygenase: dioxygenase activity and role in xenobiotic metabolism. Int. J. Biochem. 1992; 24, 255–261.
22. Bradford M. M. A rapid and sensitive method for the quantitation of microgram quantities of protein utilizing the principle of protein-dye binding. Anal. Biochem. 1976; 72, 248–254.
23. Kemal C., Louis-Flamberg P., Krupinski-Olsen R., Shorter A. R. Reductive inactivation of soybean lipoxygenase 1 by catechols: a possible mechanism for regulation of lipoxygenase activity . Biochemistry. 1987; 26, 7064–7068.
24. Hoffman P., Rauová D., Bezáková L., Obložinský M., Mikuš P. HPLC method for determination of lipoxygenase positional specific products. J. Pharm. Biomed. Anal. 2013; 84, 53–58.
25. Aggarwal B., Harikumar K. Potential therapeutic effects of curcumin, the anti-inflammatory agent, against neurodegenerative, cardiovascular, pulmonary, metabolic, autoimmune and neoplastic diseases. Int. J. Biochem. & Cell Biol. 2009; 41, 40–59.
26. Lev-Ari S., Strier L., Kazanov D., Shapiro L. M., Sobol H. D., Pinchuk I., Marian B., Lichtenberg D., Arber N. Celecoxib and curcumin synergistically inhibit the growth of colerectal cancer cells. Clin. Cancer Res. 2005; 11, 6738.
27. Basnet P., Skalko-Basnet N. Curcumin: An anti-inflammatory molecule from a curry spice on the path to cancer treatment. Molecules. 2011; 16, 4567–4598.
28. Caroll R. E., Bena R. V., Turgeon D. K., Varee S., et al. Phase II clinical trial of curcumin for the prevention of colorectal neoplasia. Cancer Prev. Res. 2011; 4(3), 354–364.
29. Gupta S. C., Prasad S., Kim J. H., Patchva S., Webb L., Priyadarsini I. K., Aggarwal B. B. Multitargeting by curcumin as revealed by molecular interaction studies. Nat. Prod. Rep. 2011; 28, 1937–1953.
30. Shureiqi I., Baron J.A. Curcumin Chemoprevention: The long road to clinical translation. Cancer Prev. Res. 2011; 4(3), 296–298.
31. Began G., Sudharshan E., Appu Rao A. G. Inhibition of lipoxygenase 1 by Phospatidylcholine micelles-bound curcumin. Lipids. 1998; 33(12), 1223–1228.
32. Skrzypczak-Jankun E., McCabe N. P., Selman S. H., Jankun J. Curcumin inhibits lipoxygenase by binding to its central cavity: theoretical and X-ray. evidence. Int°. J. Mol. Med. 2000; 6, 521–526.
33. Hajek A. R., Lindley A. R., Favoreto S., Carter R., Schleime R. P., Kuperman, D. A. 12/15-lipoxygenase deficiency protects mice from allergic airways inflammation and increases secretory IgA levels. J. Allergy Clin. Immunol. 2008; 122, 633–639.
34. Zarbock A., Distasi M. R., Smith E., Sanders J. M., Kronke G., Hardy B. L., Vietinghoff S. Improved survival and reduced vascular permeability by eliminating or blocking 12/15- lipoxygenase in mouse models of acute lung injury (ALI). J. Immunol. 2009; 183, 4715–4722.
35. Kawakami Y., Hosokawa T., Morinaka T., Irino S., Hirano S., Kobayashi H., Yoshioka A., Suzuki-Yamamoto T., Yokoro M., Kimoto M., Tsuji H., Yamashita H., Doi S., Yutani C., Kato R., Itabe H., Kanada T., Hada T., Takahashi Y. Antiatherogenic effect of guava leaf extracts inhibiting leukocyte-type 12-lipoxygenase activity. Food Chem. 2012; 131, 1069–1075.
36. Rong S., Cao Q., Liu M., Seo J., Lia L., Boudyguina E., Gebre R. K. Macrophage 12/13 lipoxygenase expression increases plasma and hepatic lipid levels and extracerebrates atherosclerosis. J. Lipid Res. 2012; doi: 10.1194/jlr. MO22723.
37. Huo Y., Zhao L., Hyman M. C., Shashkin P., Harry B. L., Burcin T., Forlow S. B., Stark M. A., Smidth D. F., Clarke S. Critical role of macrophage 12/15-lipoxygenase for atherosclerosis in apolipoprotein E-deficient mice. Circulation 2004; 110, 2024–2031.
Štítky
Farmacie FarmakologieČlánek vyšel v časopise
Česká a slovenská farmacie
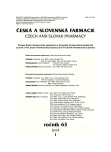
2014 Číslo 1
- Přerušovaný půst může mít významná zdravotní rizika
- Ukažte mi, jak kašlete, a já vám řeknu, co vám je
- FDA varuje před selfmonitoringem cukru pomocí chytrých hodinek. Jak je to v Česku?
Nejčtenější v tomto čísle
- Separácia enantiomérov aminokyselín metódou vysokoúčinnej kvapalinovej chromatografie
- Identifikácia a riešenie farmakoterapeutických problémov z pohľadu verejného lekárnika
- Globalizácia a jej dopady v lekárenstve SR
- Docent RNDr. Pavel Komárek, PhD. jubiluje