Complex Response of the Organism to Trauma
Authors:
Renáta Ječmínková 1; Vladimír Ječmínek 2; Jan Divák 3; Marcela Káňová 3; Ondřej Jor 3; Kamil Tomášek 3,4; Leopold Pleva 2; David Holeš 4,5
Authors place of work:
Oddělení centrálního příjmu, Fakultní nemocnice Ostrava
1; Klinika úrazové chirurgie, Fakultní nemocnice Ostrava
2; Klinika anesteziologie, resuscitace a intenzivní medicíny, Fakultní nemocnice Ostrava
3; Univerzita Komenského v Bratislave, Jesseniova lekárska fakulta v Martine
4; ÚSZS MSK Ostrava
5
Published in the journal:
Úraz chir. 26., 2018, č.2
Summary
INTRODUCTION: Severe trauma leads to a disruption of the external and internal barrier systems of the affected organism followed by a complex and highly organized reaction triggered by the release of signal molecules from the damaged tissues. These initiate both the activation of the innate immune system and the stimulation of acquired immunity and affect the nervous and hormonal systems. The effort of the organism is to maintain balance and adequacy of this complex reaction and to prevent excessive escalation of originally protective physiological processes.
OBJECTIVE: The aim of this work is to provide a comprehensive view of the complex issue of subsequent changes in the body after a severe trauma
METHODOLOGY: Review article.
CONCLUSION: More and more detailed knowledge and methods of detecting the pathophysiological processes of the body in connection with severe trauma may be the key to other possible therapeutic interventions for these injured patients.
Keywords:
trauma – stress response – nervous response – hormonal response – metabolic reaction – acquired and innate immunity
Introduction
Severe injuries are amongst the most common causes of death in the category of 1–40 year olds, and according to the World Health Organization (WHO), the cause of trauma-related deaths due to road accidents will continue to increase in the future to the estimated 5th cause of death in year 2030 [21].
Severe trauma is a major stress factor for the injured, which induced a reaction in the organism in a response at the nervous, endocrine, immune and metabolic levels. The purpose of this phylogenetically old reaction is to preserve homeostasis by: a) limiting further damage, b) mobilizing energy and c) establishing repair processes. The intensity of this response corresponds to the severity of the injury, the age and condition of the patient, but also the escalation or suppression of the initial immune and systemic inflammatory response. This is called „First Line of Defense“ or „Response to Acute Phase” [9,10].
The aim of the review article is to acquaint the reader with new findings concerning this complex response.
Methodology
Review article.
Methodology
The PubMed / Medline database (https://www.ncbi.nlm.nih.gov/pubmed) was used to search for articles dealing with the organism‘s response to trauma. A search algorithm using the „trauma“ and „response“ keywords was used. The search was limited to articles published from January 1994 to September 2018 written in English. Another source of articles were the literary references of selected publications. Also data from two Czech monographs were used.
Immune reaction
The organism‘s response to trauma is caused by stress, pain, developing shock (hypovolaemia, hypoxia, acidosis), but a very important role is also played by the release of signaling molecules from damaged, ischemic and necrotic cells - the so called alarmins, or DAMPs (damage associated molecular patterns). They are molecular patterns that are normally hidden from their own immune system and become immunologically active in connection with cell damage. They can release both a) from nuclei of damaged cells (DNA, histones, high mobility group box 1 - HMGB-1), and b) from their plasma (RNA, mitochondrial DNA) [1, 8, 22, 31, 37, 40, 41]. Once they appear out of the cell, they promote activation of natural immune cells as well as antigen-presenting cells (APCs) that are involved in the defense of the body. DAMPs are recognized soon after trauma by: a) specific membrane or cytoplasmic receptors - PRRs (pattern-recognition receptors) such as Toll like receptors (TLRs), Nucleotide-binding domain, leucine-rich repeat-containing proteins (NLRs), Receptor for advanced glycosylation end products (RAGE) and others, but also b) a serine protease system, including kinin, coagulation and complement cascades, soon after trauma. [2, 5, 6, 14, 29] DAMPs themselves are potent complement activators, leading to the rapid formation of C3a C5a [20, 23, 26].
The most comprehensively studied DAMPs in connection with the trauma-associated immune response include HMGB 1 protein, which is released into circulatory system within 30 minutes after trauma. It is a chromosomal protein involved in, for example, DNA recovery and transcription. HMGB1 is also secreted by immunocompetent cells upon encountering an infectious agent, recognizing surface markers of PAMPs (pathogen-associated molecular patterns), e.g., endotoxin (G+), lipopolysaccharide (G-). Thus, it reacts nonspecifically to any systemic inflammation with cytokine release - e.g. TNFα and IL-1. In addition, activated non-immune cells such as endothelial cells and thrombocytes can also actively produce HMGB 1 [32].
The effect of binding of HMGB1 as well as other signaling proteins on PRRs includes, but is not limited to: a) release of cytokines and chemokines from macrophages, monocytes and dendritic cells, b) activation and chemotaxis of neutrophils, c) alteration of epithelial barrier function with an increased permeability, d) increase in procoagulant platelet surface activity. Systemic inflammatory response syndrome (SIRS) develops. Two contradictory processes follow: both proinflammatory and antiinflammatory phenomena, starting from the onset of SIRS activation. Major proinflammatory cytokines include TNF alpha, IL-1, IL-2, IL-6, IL-8, interferon, PAF (platelet activating factor). Antiinflammatory cytokines include IL-4, IL-10, IL-13, prostaglandin E2, TGF β (tumor growth factor β) (Fig. 1) [32].
Stimulation of neutrophil leukocytes leads to activation of phagocytosis, release of proteases, formation of NETs (neutrolil extracellular traps) and to the formation of oxygen radicals (ROS: reactive oxygen species), among others including hydroxyl radical, superoxide radical, singlet oxygen, hydrogen peroxide. ROSs have their physiological functions: for example, they play an important role in determining the moment of resolution of inflammation as they serve as important signal molecules regulating cell growth, differentiation and apoptosis [6]. However, high ROS concentrations are detrimental to both local and remote tissues. ROS may be present both intracellularly and extracellularly. Intracellular ROS have the ability to form active inflamasomes (a group of DAMPs and PAMPs-detecting receptor proteins and providing proinflammatory protein precursor division) pro-IL-1beta and pro-IL-18. On the other hand, peroxidases contained in exosomes show extracellular activity. These may damage plasma proteins, distant organs, and blood cells after release into circulation, and may also cause premature death of mature erythrocytes (eryptosis). The resulting anemia is further contributed to by the attenuation of erythropoietin production due to inflammation and premature destruction of young erythrocytes in the so-called neocytolysis [35, 38].
In addition to the above-mentioned effects of activated neutrophils, leukocyte adhesion and systemic inflammatory response, including activation of the coagulation system occurs by the release of microvessels of the leukocytes [16, 27].
Other immunocompetent cellular action is also that of the released monocytes, which are converted to macrophages, either a) the proinflammatory phenotype M1 or b) the antiinflammatory phenotype M2. M1 macrophages further produce ROS, while M2 macrophages act at a later stage – they promote healing by releasing growth factors [25, 28].
In addition to early stimulation of both natural and acquired immunity cells, endothelial cells are activated very soon after trauma, both by primary and secondary release cytokines from neutrophil leukocytes, which are the first immune cells to translocate into tissues upon stimulation of DAMPs. The endothelial cells then amplify the immune response by further cytokine secretion and become both the trigger and the target tissue of the acting cellular mediators. They release other mediators such as the vasoconstrictor-acting thromboxane A2, they activate thrombin, and engage the coagulation and cascade complement systems with mutual links in a complex reaction. Tissue factor released from the damaged cells generates thrombin, which not only activates fibrinogen, but also endothelial cells again. Released ROS also contributes to endothelial activation. As a result, the immune response is exaggerated and the inflammation compartmentalization is lost. The result of the so-called cytokine storm is the alteration of Na + -K + ATPase with electrophysiological membrane dysfunction [15].
In case of severe trauma, especially in the presence of hemorrhagic shock or in case of need of a prolonged surgical intervention, both traumatic coagulopathy and so-called endothelopathy and complementopathy, called trauma-induced alteration to immune and inflammatory responses, soon develop [5, 17]. The effect of ischemia-reperfusion also contributes to this. The result is an escalated immune, coagulation and ROS response with microangiopathy and cellular barriers fault, which may contribute to the further formation and release of DAMPs and the formation of circulus vitiosus. The clinical manifestation of this harmful immune response is infection, sepsis or MODS [40] (Fig. 2).
In addition to the aforementioned complications, patients critically affected after a severe trauma are endangered in the longer term (14 days or more after an injury) even by the so-called PICS (persistent inflammation, immunosuppression and catabolism syndrome), which is associated with continued catabolism and signs of congenital and acquired immunosuppression [12] (Fig. 3). There is reduced cytokine production, loss of monocyte-macrophage function, persistent increase in MDSCs (myeloid-derived suppressor cells), and a decrease in the count and weakening of the function of effector T-lymphocytes. This syndrome correlates with poorer wound healing, infections, sarcopenia, prolonged recovery and increased mortality [12, 22].
Therefore, the ability of the body to establish a balance for and the anti-inflammatory condition or, on the contrary, the persistent imbalance of these complex immune processes may result in a significant contribution to the course of recovery or the development of post-traumatic complications.
Neuroendocrine reactions
The activation of the sympathetic nervous system upon a severe trauma is immediate, by stimulating the postsynaptic fibers of the sympathetic ganglia with noradrenaline release, and by stimulating preganglionic sympathetic fibers with adrenaline release in the adrenal medulla. The rapid effect takes place through alpha and beta receptors with a positive inotropic, chronotropic, vasodilatory (brain, heart, skeletal muscle), vasoconstrictive (splachnic, kidney, skin) and metabolic effects. This involves the rapid mobilization of energy reserves with glucose availability through increased glycogenolysis, increased glucagon secretion, reduced insulin secretion, stimulation of lipolysis, stimulation of the reninangiotensin-aldosterone system, and activation of the hypothalamic hypophyseal axis (HPA).
In contrast, activation of the parasympathetic nervous system has an antiinflammatory effect. The afferent vagal nerve fibers, after stimulation with both cytokines and PAMPs, lead to the brain stem and in the way of efferent fibers stimulate splenic sympathetic fibers in the celiac plexus, which via the beta2-adrenergic receptor release acetylcholine from T lymphocytes that contain functional choline acetyltransferases. ACh reacts with nicotinic receptors (α7nAChRs) of macrophages, inhibits NF kappa B and subsequently the cytokine synthesis and release incl. TNF alpha [13, 30].
This cholinergic anti-inflammatory pathway appears to be critically involved in the control of systemic inflammation [27]. The source of acetylcholine acting in this way are not only the nerve endings but also the immune cell [7]. In summary, this regulatory pathway has been termed the „cholinergic anti-inflammatory pathway” [19].
The hypotalalamus-hypophysis axis is also stimulated by stress, pain, but also by the presence of released IL-1, IL-6, TNF alpha, where the paraventricular nucleus of the hypothalamus is initiated to secrete CRH (corticotropin-releasing hormone with stimulation of adenohypophysis hormone secretion) - via POMC (proopiomelanocorticotropin) to release ACTH (adrenocorticotropic hormone) followed by glucose and mineralocorticoid secretion. Among other things, it stimulates the secretion of endorphins and lipotropins, secretion of STH (somatotropin), prolactin, TSH (thyreotropin), FSH (follicle stimulating hormone), LH (luteinizing hormone). In addition, hypothalamus also enusre direct neuronal secretion of adiuretin from neurohypophysis.
The main stress hormones include cortisol, which is released after de novo synthesis by the adrenal cortex, and its level is likely to correlate with the degree of burden imposed upon the body. However, low levels of cortisol may be present as a reflection of the relatively inadequate response of the organism to stress, called „relative adrenal insufficiency.“ The rapid increase in circulating cortisol (already in the order of minutes after trauma and peaking in 4–6 hr) has multiple effects. The metabolic effect consists in an increased blood glucose, increased insulin resistance, proteolysis, and lipolysis. Other effects are the permissive effect of catecholamines with increased inotropy, vasoconstriction, anti-inflammatory effects include thymus involution, cellular immunity suppression mediated by decreased cytotoxic T-lymphocyte and NK-cell function, T-cell blastogenesis. Also, glucocorticoids inhibit neutrophil superoxidase activity, suppress chemotaxis, and normalize the signal mechanism of apoptosis, but retain the phagocytic function of neutrophils [18, 36]. They further reduce prostaglandin and leukotriene formation by blocking phospholipase A2. Furthermore, they have antiallergic effects, cortisol also increases bone resorption, decreases calcium absorption in the intestine, and mineralocorticoid effects may also occur with persistent high blood levels.
Somatotropin, in addition to its metabolic effect with the support of gluconeogenesis, anti-insulin and proteoanabolic effects, affects immunity by promoting antibody production, collagen, increased IL-2 synthesis, NK cell formation, macrophages and cytotoxic T lymphocytes, and stimulates erythropoietin generation.
Prolactin inhibits glucose uptake into cells, has an immunomodulatory effect by stimulating T cells.
Total testosterone, free testosterone and dihydrotestosterone decrease very soon after trauma only with a gradual tendency to adjust. Deficiency is manifested by impaired physiological function of protein synthesis stimulation.
The second major axis after HPA is the renin-angiotensin-aldosterone (RAAS) system. It is stimulated by sympathetic, renal hypoperfusion and decreased sodium concentration in macula densa. Renin converts angiotensinogen to angiotensin I, which is converted by ACE (angiotensin converting enzyme) to angiotensin II with an effect on aldosterone release from the adrenal glomerulosis zone, further releasing the ACTH and antidiuretic hormone by stimulating the hypothalamus. It is a very potent vasoconstrictor. Aldosterone increases the reabsorption of sodium in the kidney distal tubule with a volumic effect.
In addition to angiotensin II, the antidiuretic hormone is also released by adrenaline, pain and hyperglycaemia. Above all, it has a volumic effect by increasing water resorption in the kidney collecting duct, but it also has an endocrine effect, when it stimulates the release of POMC through CRH (corticoliberin). Its level remains elevated for up to 1 week from the insult depending on the degree of injury and time of hemodynamic instability. As a potent vasoconstrictor of the splachnic bed it may contribute to the development of intestinal ischemia and dysfunction of the bowel barrier function [4].
During the reaction of the organism to trauma (but also hemorrhagic shock, ischemia, hypoxia), both neuroendocrine and immune systems „communicate“. They share the same information molecules (hormones, neurotransmitters, neuropeptides, cytokines and their receptors).
Direct neuro-immune communication between hormones (CRH, ATH, prolactin, TRH, vasopressin) and lymphocyte production and activation is described [3, 18, 32, 33]. Not only due to released lymphokines, but also the lymphocytes themselves are able to synthesize neuroendocrine peptides such as ACTH, gamma-endorphinrelated substances, immunoreactive growth hormone and thyreotropin. These peptides can then modulate the lymphocyte and other immunocompetent cell responses to regulate the count of new B lymphocytes produced in the bone marrow [24]. There is evidence of production of opoid peptides, noradarenaline and adrenaline, both by lymphocytes and macrophages [11].
Thanks to the numerous feedbacks between the neuroendocrine and immune system, referred to as the sixth sense, these links affect the function of many organs. The fastest clinical responders are the brain with the development of a delirious state. The success in restoring balance of the pro-inflammatory and anti-inflammatory events with respect to the severity of trauma implies whether tissue regeneration or the development of MODS (multiple organ dysfunction syndrome) takes place.
Metabolic reaction
Metabolic response to stress makes part of an adaptive response in an effort to survive trauma. Due to activated replacement metabolic pathways, especially catabolic hormone production and insulin/anabolic peripheral resistance, energy is obtained from alternative sources. The survival tax will be paid by the body primarily by muscle loss.
As early as in the 1940s, dr. Guthbertson presented the main stages of the state of shock. These are: ebb phase - early shock with metabolic attenuation, followed by flow phase or catabolic phase, followed by a different length of recovery and anabolic phases [39].
In the ebb phase lasting 24–48 hour after insult, the action of cytokines, eicosanoids, ROS, and other local mediators results in the suppression of metabolic activity, reduced oxygen consumption, decreased cardiac output, and increased peripheral vasoconstriction. In response to washed out catecholamines and hormones, insulin resistance develops, blood glucose increases, and lipolysis is activated. Due to persistent hypoxia, metabolic acidosis develops with endothelial disorder, deteriorated O2 transport to tissue cells, and accumulation of metabolites with potential cell failure [35].
In the flow phase, hyperkinetic circulation dominates with increased blood perfusion of the liver, kidneys and injured areas, increased O2 consumption, energy substrates, peripheral glucose utilization increases, gluconeogenesis increases, which is the first metabolic change after trauma. Fatty acids are also increasingly mobilized. Much of the increased energy demand is proportional to the severity of the trauma and asserts itself by the compensation of damaged membrane potentials by the action of cytotoxins and endotoxins. It is assumed that up to 40 of the energy demand is used to cover the energy of ion pumps and transport processes [28].
Significant muscle catabolism is dominant in the hypermetabolic phase. The released amino acids serve both as a source for gluconeogenesis and for the synthesis of acute phase proteins. Gluconeogenesis is vitally important as an energy source of glucose for neurons, leukocytes, macrophages, and cells of compromised tissues - hypoxic, inflammatory, and healing areas where mitochondrial respiration is not possible. The source is not only in amino acids, but also lactate (Corri cycle) and glycerol. Glycogen reserves are consumed within a short time interval. The action of hormone sensitive lipase releases fatty acids (FAs) and glycerol into the circulation. FAs can be re-esterified to triglycerides, that with their partial oxidation in the liver, which can result in fatty liver infiltration, especially in the presence of a higher dose of continuous glucose /limit in adults of 4mg/kg/min/ or in diabetic, obese patients and septic patients [34].
MUDr. Renáta Ječmínková
Zdroje
- Beghard, F., Hubert-Lang, M. Polytrauma – pathophysiology and management principles. Langenbecks Arch Surg. 2008, 393, 825–831. ISSN 1435-2451
- Billiar, TR, Vodovitz, Y. Time for trauma imunology. PLoS Med. 2017, 14, e 1002342. ISSN 1544-9173
- Brian, S., Zuckerbraun et al. Shock. In: Schwartz´s principles of surgery. New York: McGraw-Hill Medical, 2015, 109–134. ISBN 978-0-07-180092-1
- Brunicardi, FC. Schwartz´s Principles of Surgery. 10th ed. New York: McGraw-Hill Medical, 2015. 2069 p. ISBN 978-0-07-180092-1
- Burk, AM. et al. Early complementopathy after multiple injuries in humans. Shock. 2012, 37, 348–354. ISSN 1073-2322
- Ceki, C., Linden, J. Purinergic regulation of the immune system. Nat Rev Immunol. 2016, 16, 177–192. ISSN 1474-1776
- Dash, PK et al. Activation of alpha7 cholinergic nicotinic receptors reduce blood-brain barrier permeability following experimental traumatic brain injury. J Neurosci. 2016, 36, 2809–2818. ISSN 0270-6474
- Davis, GE., Bayless, KJ., Davis, M. et al. Regulation of tissue response by the exposure of matricryptic sites within extracellular matrix molecules. Am J Pathol. 2000, 156, 1489–1498 ISSN 1432-0533
- DINARELLO, CA. Interleukin I and the pathogenesis of the acute phase response. N Engl J Med. 1984, 311, 1413–1418. ISSN 1310-1314
- BAUMANN, H., GAULDIE, JH. The acute phase response. Immunol Today. 1994, 15, 74–80. ISSN 0167-5699
- DOLEČEK, R. et al. Endokrinologie traumatu. Praha : Maxdorf, 2016. 215 s. ISBN 978-80-7345-484-5
- Engler, KL, Rudd, ML, Ryan, JJ. et al. Autocrine actions of macrophage-derived catecholamines on interleukin 1 beta. J Neuroimmunol. 2005, 160, 87–91. ISSN 0165-5728
- Frith, D. et al. Definition and drivers of acute traumatic coagulopathy: clinical and experimental investigation. J Tromb Haemost. 2010, 8, 1919–1925. ISSN 1212-0383
- Gentile, LF. et al. Persistent inflammation and immunosuppression: a common syndrome and new horizon for surgical intensive care. J Trauma Acute Care Surg. 2012, 72, 1491–1501. ISSN 2163-0755
- Gnater, MT. et al. Role of the alternative pathway in the early complement activation following major trauma. Shock. 2007, 28, 29–34. ISSN 1073-2322
- Griffiths, H., Gao, D., Pararasa, C. Redox regulation in metabolic programming and inflammation. Redox Biology. 2017, 12, 50–57 ISSN 2213-2317.
- Hayden, S., Albert, T., Watkins, T. et al. Anemia in critical illness. American Journal o Respiratory and Critical Care Medicine. 2012, 185,1049–1057. ISSN 1073-449X
- Chen, W. et al. Cytokine cascades induced by mechanical trauma injury alter voltage-gated sodium channel activity in intact cortical neurons. J Neuroinflammation. 2017, 14, 73. ISSN 1742-2094
- Igors, I., Maris, M., Viesturs, B. Stress response to surgery and possible ways of its correction, Proc Latvian Acad Sci. 2012, sekce B, 66. ISSN1407-009X
- Kawashima, F. 2004, Expression of non-neuronal acetylcholine in lympfocytes and its contribution to the regulativ of immune function. Front Biosci. 2009, 9, 908–910. ISSN 1093-9946
- Lord, AB, Martin, M., Flierl, MA. Early complementopathy after multiple injurie in humans. Shock. 2012, 37, 348–354. ISSN1073-2322
- Lord, JM. et al. The systemic immune response to trauma: an overview of pathophysiology and treatment. Lancet. 2014, 384, 1455–1465. ISSN 0140-6736
- Lung, MH., Kambria, JD., Ward, PA. Innate immune responses to trauma. Nat Imunol. 2018, 19, 327-341. ISSN 1529-2908
- MHuber, L., Kovkun, A., Ignatius, A. The role of complement in trauma and fracture healing. Semin Immunol. 2013, 25, 73–78. ISSN 1044-5323
- Maren, S., Fragala, W., Kraemer, J. et al. Neuroendocrine-Immune Interactions and Responses to Exercise. Sports Med. 2011, 41, 8, 621–639. ISSN 1044-5323
- Matijevic, N. et al. Microvesicle phenotypes are asssociated with transfusion requirements and mortality in subjects with severe injuries. J Extracell. 2015, 4, 29338. ISSN 2001-3078
- MDNeher, M., Weckbach, S., Flierl, MA. et al. Molecular mechanism of inflammation and tissue injury after major trauma-is complement the „bad guy“? Journal of Biomedical Science. 2011, 18, 90. ISSN 1423-0127
- Mitchell, TA. et al. Traumatic hemothorax blood contains elevated levels of microparticles that are prothrombotic but inhibic platelet aggregation. Shock. 2017, 47, 680–687. ISSN 1073-2322
- Mittal, M., Siddiqui, M., Tran, K. et al. Reactive oxygen species in inflammation and tissue injury. Antioxidants and Redox Signaling. 2014, 20, 1126–1167. ISSN 1523-0864
- Netea, MG. et al. A guiding map for inflammation. Nat Immunol. 2017, 18, 826–831. ISSN 1529-2908
- Pavlov, VA., Tracey, KJ. Neural regulation of immunity: molecular mechanisms and clinical translation. Nat Neurosci. 2017, 20, 156–166. ISSN 1097-6256
- Qiang, X. et al.Cold inducible RNA-binding protein (CIRP) triggers inflammatory response in hemorrhagic shock and sepsis. Nat Med. 2013, 19, 1489–1495. ISSN 1078-8956
- Siobhan, A. Corbett, SL. Systemic Response to Injury and Metabolit Support. In: Schwartz´s principles of surgery. New York: McGraw-Hill Medical, 2015, s. 13–63. ISBN 978-0-07-180092-1
- Smith, EM. Neuropeptides as signal molecules in common with leucocytes and the hypothalamic-pituitary-adrenal axis. Brain Nebav Immun. 2008, 22, 3–14. ISSN 0889-1591
- Sobotka, L., Soeters, PB. Metabolic response to injury and sepsis. In: Basics in clinical nutrition. Praha: Galén, 2011, s. 197–202. ISBN 978-80-7262-821-6
- Tafani, M., L.Sansone, F. Limana, T. et al. The interplay of reactive oxygen species, hypoxia, inflammation and sirtuins in cancer initiations and progression. Oxidative Medicíne and Cellular Longevity. 2016, 20, 1–18. ISSN 1942-0994
- Tracey, KJ. Physiology and immunology of the cholinergic anti-inflammatory pathway. J Cli Invest. 2007, 117, 289–296. ISSN 0021-9738
- Trebichavský, I. Vznik a ukončení zánětu. Živa. 2017, 65, 2–4. ISSN 0044-4812
- Walsh, TS, Saleh, EE. Anaemia during critical illness. Br J Anaesth. 2006, 97, 278–279. ISSN 0007-0912
- ZADÁK, Z. Výživa v intenzivní péči. Praha : Grada Publishing a.s., 2008. 544 s. ISBN 978-80-247-2844-5
- Zhang, Q. et al. Circulating mitochondrial DAMPs cause inflammatory response to injury. Nature. 2010, 464, 104–107. ISSN 1476-4687
- Zhao, H., Kilgas, S., Alarm, A. et al. The role of extracellular adenosine triphosphate in ischemic organ injury. Crit Care Med. 2016, 44, 1000–1012. ISSN 1530-0293
Štítky
Chirurgie všeobecná Traumatologie Urgentní medicínaČlánek vyšel v časopise
Úrazová chirurgie
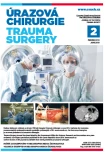
2018 Číslo 2
- Metamizol jako analgetikum první volby: kdy, pro koho, jak a proč?
- Neodolpasse je bezpečný přípravek v krátkodobé léčbě bolesti
- Léčba akutní pooperační bolesti z pohledu ortopeda
Nejčtenější v tomto čísle
- Results of Achilles tendon rupture repair. Classical open approach versus percutaneous suture
- Complex Response of the Organism to Trauma
- Addressing of trochanteric femoral fractures using a revision femoral stem with a cervicocapital prosthesis – coxofemoral by-pass
- Abdominal trauma and wandering spleen - case report