INDUCTION SENSORS FOR MEASUREMENT OF VIBRATION PARAMETERS OF ULTRASONIC SURGICAL WAVEGUIDES
Authors:
D. Stepanenko 1; V. Minchenya 1; A. Avtushko 1; K. Bogdanchuk 1; E. Dlusskaya 1; I. Yanovich 1; T. Stepanenko 2
Authors‘ workplace:
Belarussian national technical university, Minsk, Republic of Belarus
1; Research institute of automation means, Minsk, Republic of Belarus
2
Published in:
Lékař a technika - Clinician and Technology No. 2, 2012, 42, 11-14
Category:
Conference YBERC 2012
Overview
The article describes design, fabrication technology and results of experimental studies of induction sensors for measurement of vibration parameters of ultrasonic surgical waveguides. The developed sensors are based on effect of inverse magnetostriction and have simple and miniature design. They have improved locality of measurements and this provides possibility to study distribution of vibration parameters along the length of the waveguide. The described sensors can be used during design and testing of ultrasonic waveguides for minimally-invasive surgery and as feedback sensors in ultrasonic surgical devices.
Keywords:
ultrasonic waveguide, induction sensor, inverse magnetostriction
Introduction
Measurement of parameters of ultrasonic vibrations, particularly, amplitude of mechanical stresses, is actual practical problem, because ultrasound is widely used in engineering and medicine. At present there is a number of non-contact sensors for measurement of vibration parameters of ultrasonic waveguides including fibreoptic sensors, laser Doppler vibrometers (LDVs), inductive and induction sensors. Direct measurement of amplitude of ultrasonic vibrations can be implemented by means of microscope and this method is used for calibration of the above mentioned sensors. The main drawbacks of fibre-optic sensors and LDVs are their high cost, complexity of design and signal processing. The most easily implemented sensors are inductive and induction ones. For example, the authors previously described design of induction sensor for measurement of vibration amplitude of ultrasonic surgical waveguides and application of this sensor for control of amplitude-frequency response functions of such waveguides [1]. As a special case of induction sensors one can consider sensors based on effect of inverse magnetostriction. This effect, also known as Villari effect, consists in alteration of magnetization of ferromagnetic materials under the action of mechanical stresses. Application of time-varying stresses to the material will create alternating magnetic field, which can be detected by means of induction coil. This method of detection of ultrasonic stresses was for the first time described by Langenecker [2]. Effect of inverse magnetostriction is widely used in nondestructive evaluation and for study of magnetic properties of materials. For example, Hatafuku describes application of inverse magnetostriction induced by ultrasonic stresses for determination of effective magnetic field in ferromagnetic bars [3]. The main drawback of measurement of parameters of ultrasonic vibrations by means of induction sensors on the basis of effect of inverse magnetostriction is nonlocality of measurement. This non-locality is manifested in the fact that electromotive force (EMF) induced by alternating magnetic field in long helical induction coil is determined by mechanical stresses acting in all cross-sections of the waveguide encircled by loops of the coil. This makes impossible determination of stresses in specific cross-section. This article considers design, manufacturing technology and results of experimental studies of sensors of ultrasonic stresses based on effect of inverse magnetostriction with improved locality of measurements as well as possibility of application of these sensors for control of vibration parameters of ultrasonic surgical waveguides.
Design and fabrication methods of the sensors
Locality of measurements using induction sensors on the basis of effect of inverse magnetostriction can be improved by means of application of flat (spiral) induction coils. This idea was used for development and fabrication of three types of induction sensors with flat coils. In two of these sensors coil was fabricated by means of single-layer spiral winding of insulated wire conductor, and in the third sensor it was produced using electrochemical etching of thin-film conductive material (foil). Photographs of appearance of the developed sensors are presented in the Fig. 1.
Sensor of the first type was fabricated using copper wire with diameter 0.7 mm coated with insulating lacquer. Wire was wound on non-ferromagnetic core in the form of short-length polystyrene tube. For ensuring single-layer packaging of wire winding of coil was implemented between two plates made of organic glass. Fixed clearance with value equal to diameter of wire was set between the plates. Core was inserted with small interference into the openings made in the centres of the plates. Length of core was chosen to be slightly smaller than sum of thicknesses of the plates and clearance between them. Clearance was set by inserting single turn of wire between the plates with their subsequent fixation by means of screw passing through the opening of core, washer and nut. Central turn of wire was fed from the sensor body through axial slot made in opening of one of the plates and radial cut in the washer. After winding of the necessary number of coil turns fixation screw was removed from the opening of core, and plates were kept in compressed state by means of screws inserted in openings circumferentially arranged at the outer edges of the plates. Utilization of plates made of transparent material enabled visual inspection of winding, particularly, presence of close packaging of coil turns.
In the sensor of the second design coil was wound using copper wire with diameter 0.06 mm. This enabled miniaturization of sensor (dimension with electronic components is Ø40 mm × 3 mm). Special feature of the sensor consists in the presence of built-in signal amplifier with low supply power. Power supply of amplifier is implemented using auxiliary coil, which transforms alternating magnetic field arising during vibration of the waveguide into supply voltage. In other words, power supply of amplifier is implemented using principle of magnetostrictive harvesting of vibration energy [4].
Sensor of the third type was fabricated using foilcoated cloth laminate with thin-film copper coating. Foil was mechanically polished, after that it was coated with mask protecting conductive tracks of coil from etching. In this work we generated mask using simplified technology applied by radioamateurs for production of homemade circuit boards and based on application of laser printer. First of all mirror image of mask was created. This task was implemented using AutoCad software and specially developed macro in VBA programming language for drawing of spirals. Image of mask was transferred onto paper carrier (tracing paper) using laser printer. After that toner powder was transferred from paper carrier onto copper coating of cloth laminate substrate using heating element with temperature about 180° C. Under uniform pressure and heating toner powder strongly adheres to the copper coating, and tracing paper can be removed by means of water. Drawback of the method consists in spreading of toner powder as a consequence of melting, resulting in spreading and non-uniform width of tracks of the mask. Under excessive pressure and heating spreading can result in formation of bridges between adjacent tracks of the mask. After etching these bridges give origin to cross-track short-circuiting. For avoidance of this problem one needs to remove bridges by means of needle or other sharp tool. Etching of copper was implemented using water solution of chloride of trivalent iron (FeCl3). Duration of etching was about 2.5 hours, however, it can be substantially reduced by heating solution up to 50° C. It should be noted that large duration of etching is not a critical factor from the view of productivity since it is possible to use batch processing enabling simultaneous fabrication of large quantity of sensors. Under insufficiently strong adherence of toner powder to the substrate small underetching of tracks was observed, appearing as matt areas of the surface in the Fig. 1c. After etching toner powder was washed away from the substrate using acetone, drilling of central opening and soldering of signal wires were carried out. For protection of harmful external physical and chemical conditions tracks of the sensor can be coated with layer of insulating lacquer. In industrial conditions fabrication of foil sensors can be implemented using foil-coated polyimide films used in production of flexible circuit boards and having thickness down to 45 μm. Small thickness of the films provides potential possibility for creation of compact multilayer sensors, for example consisting of one or several layers of signal-detecting coils and one or several layers of energy-harvesting coils. Such sensors will preserve locality of measurements and posses enhanced sensitivity and efficiency of energy harvesting. Fabrication of coils can be implemented by means of photolithography, laser ablation or Focused Ion Beam (FIB) milling as well as by means of electrochemical etching with application of mask produced by direct printing on the substrate [5]. Above-listed methods provide possibility of fabrication of coils with precise dimensions and very small features (small pitch of conductive tracks).
Study of operational characteristics of the sensors
Experimental studies of the developed sensors were implemented on wire ultrasonic waveguides and bars made of austenitic steel 316L. EMF induced in the sensor coil can be determined from Faraday law:
where Φ(t) is time-varying magnetic flux traversing coil; Φ0 is amplitude of magnetic flux; f is vibration frequency. It is supposed that magnetic flux varies in harmonic law:
As it follows from Eq. (1), amplitude of EMF is proportional to vibration frequency. For high vibration frequencies (20-30 kHz) this provides possibility to detect signal with sufficiently large amplitude even for very small variations of magnetization.
Testing of linearity of the developed sensors was implemented by measuring amplitude of stresses in longitudinally vibrating rigid bar with diameter 4 mm. Signal observed in all sections of the waveguide had harmonic form and this proves linearity of the sensors. Distribution of stress amplitude along the length of the waveguide is in agreement with theoretically predicted sinusoidal distribution (Fig. 2). Maximum amplitude of the signal is observed in nodal points of vibratory displacements corresponding to antinodes of mechanical stresses.
In the case of flexible waveguides with diameter of input section 2 mm waveguide will also perform flexural vibrations besides longitudinal ones. As a result of nonlinear mechanical interaction of these two vibration modes output signal of the sensors will become non-harmonic. Spectral analysis of the signal can provide information on amplitudes of longitudinal and flexural components of vibration. Spectral analysis can be implemented by means of oscillograph with functions of mathematical processing of signals. Mathematical processing can be also performed using standard mathematical software, for example, MathCad. In this work we used photographic registration of the signal with subsequent software processing of image in MathCad. Image was saved in BMP format after that we extracted green channel of the image and represented intensities of its pixels in the form of matrix (selection of color channel is related to the green color of cathode-ray-tube phosphor in oscillograph). Extraction of signal curve from the background of image was implemented using threshold segmentation [6]. Example of the image with superimposed segmented curve is presented in the Fig. 3.
Spectral analysis of the given signal showed prevalence of fundamental and third harmonics. Fundamental harmonic presumably corresponds to longitudinal component of vibration, and third harmonic – to flexural component.
Longitudinal and flexural components can be separated by means of bias magnetization and this will be objective of the future studies. In the presence of uniform bias magnetic field (for example, created by Helmholtz coils) sensor will detect only component of stresses related to longitudinal deformation, and in the presence of asymmetric bias field created by permanent magnets it will detect only stress component related to transverse (flexural) deformation [7]. In the presence of asymmetric bias field induction detection of flexural deformation becomes possible even for nonferromagnetic materials with high electric conductance, for example, aluminum [8]. Such possibility is based on reversed Lorentz-force mechanism used in electromagnetic acoustic transducers (EMATs): movement of conductive material in magnetic field under the action of external force results in generation of eddy current inducing EMF in detection coil.
In the future it is also planned to calibrate developed sensors. Calibration can be implemented using direct measurement of amplitude of vibratory displacements by means of microscope with account for theoretical relations between vibratory displacements and stresses. It is also possible to measure amplitude of stresses by means of tensoresistive transducers [9].
Another interesting problem is experimental modal analysis of waveguides. Such analysis demonstrated in other works on induction sensors [10] will be especially useful for explanation of nonlinear mechanical interactions between longitudinal and flexural vibrations.
The developed sensors can be used for study of vibratory processes in ultrasonic surgical waveguides during their development and testing and as feedback sensors in ultrasonic surgical devices with adaptive keeping of resonance.
Conclusions
In conclusion, we developed three types of inductive sensors for measurement of mechanical stresses in ultrasonic surgical waveguides and technology of fabrication of these sensors. The developed sensors have good linearity, improved locality of measurement, simple and compact design. They can be applied in research laboratories during design and testing of ultrasonic waveguides for minimally-invasive surgery as well as in surgical ultrasonic devices as feedback sensors. We also described future directions in experimental studies and practical applications of the developed sensors.
Acknowledgements
The authors would like to acknowledge Roustam Asimov and specialists of Sensotronica Co. Ltd., Resident of the High Technologies Park for assistance in development of the sensor with built-in amplifier.
Dmitry Stepanenko, Ph.D.
Department of construction and production of instruments
Instrument-making faculty
Belarussian national technical university
65 Nezavisimosty Ave.,
220027 Minsk, Republic of Belarus
E-mail: stepd@tut.by
Phone: +375 17 2939101
Sources
[1] Stepanenko, D. et al. Computer modelling and experimental studies of wire ultrasonic waveguides for minimally-invasive surgery. Lékař a Technika, 2010, vol. 40, No. 2, p. 1-5.
[2] Langenecker, B. Inverse magnetostrictive effect of ultrasonic stress waves in steel. Journal of Acoustical Society of America, 1965, vol. 38, p. 234-238.
[3] Hatafuku, H. Estimation of effective magnetic field in a nickel rod by the magnetoacoustic effect. Journal of Magnetism and Magnetic Materials, 2002, vol. 239, p. 94-96.
[4] Wang, L., Yuan, F.G. Vibration energy harvesting by magnetostrictive material. Smart Materials and Structures, 2008, vol. 17, article 045009.
[5] Rapid Microsystem Prototyping – Stanford NEMS. http://nems.stanford.edu/mediawiki/index.php/Rapid_Microsyst em_Prototyping
[6] Handbook of medical imaging processing and analysis. Editorin-chief I.N. Bankman. – San Diego, San Francisco, New York, Boston, London, Sydney, Tokyo: Academic Press, 2000. – 893 p.
[7] Lee, H., Kim, Y.Y. Wave selection using a magnetomechanical sensor in a solid cylinder. Journal of Acoustical Society of America, 2002, vol. 112, p. 953-960.
[8] Kim, Y.Y., Han, S.W. Magnetic sensor for noncontact modal testing of a nonferromagnetic cylinder. Modal Analysis Conference 2007 (IMAC-XXV): A Conference and Exposition on Structural Dynamics, Orlando, USA, February 19-22, 2007.
[9] Lamport, H., Zinsser, H.H. Strain gauge measurement of output of magnetostrictive ultrasonic transducer – pitfalls of optical measurement. Journal of Acoustical Society of America, 1950, vol. 31, p. 435-438.
[10] Han, S.W., Kim, Y.Y. Magnetostrictive sensor applications for the modal testing of insulated pipe. The 32nd International Congress and Exposition on Noise Control Engineering, Seogwipo, Korea, August 25-28, 2003, p. 397-403.
Labels
BiomedicineArticle was published in
The Clinician and Technology Journal
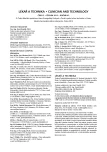
2012 Issue 2
Most read in this issue
- MECHANICAL MODEL OF THE CARDIOVASCULAR SYSTEM: DETERMINATION OF CARDIAC OUTPUT BY DYE DILUTION
- MATLAB AND ITS USE FOR PROCESSING OF THERMOGRAMS
- VENTILATOR CIRCUIT MODEL FOR OPTIMIZATION OF HIGH-FREQUENCY OSCILLATORY VENTILATION
- SETTING EMG STIMULATION PARAMETERS BY MICROCONTROLLER MSP430