NONINVASIVE SYSTEM FOR LOCALIZATION OF SMALL REPOLARIZATION CHANGES IN THE HEART
Authors:
Olena Punshchykova 1,3; Peter Kneppo 1; Milan Tyšler 1,2
Authors‘ workplace:
Faculty of Biomedical Engineering, Czech Technical University in Prague, Kladno, Czech Republic
1; Institute of Measurement Science, Slovak Academy of Science, Bratislava, Slovakia
2; Intercollegiate Faculty of Medical Engineering, National Technical University of Ukraine
“Kyiv Polytechnic Institute”, Kyiv, Ukraine
3
Published in:
Lékař a technika - Clinician and Technology No. 2, 2012, 42, 7-10
Category:
Conference YBERC 2012
Overview
Novel noninvasive method for localization of areas of myocardium with changed repolarization that can lead to life threatening cardiac arrhythmias is presented. Method is based on multichannel ECG measurement, body surface potential mapping and solving the inverse problem of electrocardiology to find one or two dipoles representing the pathological sources in the heart. Body surface potential mapping system ProCardio-8 using up to 144 active electrodes was used for multichannel ECG measurement. For the inverse calculations, inhomogeneous model of the shape of thorax with lungs and ventricles including cavities was used. Ability of the system to identify selected cardiac pathologies is demonstrated on cases with one or two ischemic foci. In this paper, analysis of existing system and algorithms for cardiac pathology localization is given in detail. Further software development will include ventricular premature beat foci localization.
Keywords:
multichannel ECG recording, body surface potentials, inverse problem of electrocardiography, repolarization changes, arrhythmia
Introduction
According to the European Heart Rhythm Association White Book 2011, in 2009 the proportion of deaths resulting from cardiovascular diseases was 50% in the Czech Republic and 65% in Ukraine [1]. Therefore it is vital to decrease these numbers using new diagnostic and treatment methods.
Necessary precondition for the use of advanced diagnostic methods enabling precise cardiac pathology localization in various cardiovascular diseases is availability of quality high resolution measurement of electrocardiograms.
Diagnostic information contained in body surface potential maps and acquired from multichannel ECG signals is much more detailed than that obtained from standard 12-lead ECG or Frank VCG lead system.
For high quality measurement of required ECG signals special multichannel electrocardiographic system ProCardio-8 was developed [2].
Material and Methods
Body surface potential mapping (BSPM) is a technologically advanced method that helps in detailed diagnostics of various cardiovascular diseases. Using BSPM for localization of areas with changed repolarization can significantly improve the understanding of electrophysiological processes in the heart in different clinical cases. The procedure is noninvasive and non-fluoroscopic and can provide highly useful diagnostic information without substantially loading the patient. In addition, its safety, possibility of repeated examinations and their automation gives BSPM more important advantages in comparison to other diagnostic procedures.
BSPM is based on multichannel measurement of body surface cardiac potentials using special multichannel electrocardiograph. Detailed registration of the surface cardiac potentials requires high number of sensing electrodes accurately placed on the thorax.
BSPM is one of the several cardiac mapping methods. “Cardiac mapping” is a wide term which also covers endocardial and epicardial mapping. Endocardial mapping is performed with catheters, which are inserted into heart cavities and navigated using fluoroscopy. Inserted catheters sequentially record endocardial electrograms and enable to identify temporal and spatial distributions of electrical potentials generated by the myocardium during normal and abnormal rhythms.
In contrast to the above mentioned two methods of cardiac mapping, noninvasive assessment of the electrical state of the heart by solution of the inverse problem of electrocardiography requires high quality surface ECG measurement with correct and precise electrode positioning and an appropriate model of the patient torso volume conductor. This model can be either some simplified approximation of a real torso, or can be precisely derived from CT or MRI scans of the patient torso what considerably increases accuracy of the method. The variety of cardiovascular pathologies that can be identified using such noninvasive inverse procedure includes ischemia, WPW, ventricular ectopy and other arrhythmias [4].
Multichannel ECG mapping system ProCardio-8
In order to record and analyse high quality multichannel ECG recordings, a special system ProCardio-8 was designed. This device consists of a set of active electrodes (up to 144), a data acquisition system and a hosting personal computer. Rechargeable Li-ion battery power supply with advanced power management is used for achieving excellent recordings quality and possibility of its using for one working day without replacement or recharging.
The data acquisition system is placed in a patient terminal box and connected via USB cable to the host personal computer. Its small geometric dimensions (14 × 19 × 20 cm) minimize capacitive coupling with external environment. The data acquisition system is modular and built from up to 9 measuring boards that are plugged into a motherboard. The system can be configured to record ECG signals simultaneously from 64 to 144 electrodes. Each measuring board records 16 ECG signals. One of the boards is configured as the reference board and is used for recording of 3 signals from the limb electrodes R, L and F. The mean value from the limb electrodes - the potential of the Wilson's central terminal (WCT) that is commonly used as the reference for all unipolar leads - is generated in a resistor network as the 4th signal from the board. Resting 12 channels on the board can be used for signals from additional chest electrodes.
The reference board also contains additional circuitry for the common mode sense electrode (CMS) and the driven right leg (DRL) electrode that provides active grounding of the patient and reduces the common mode voltage. DRL circuit also limits the current through the patient body to 50 μA. For patient protection in case of possible electrical defects, additional protection circuit is used that generates a power-down signal in case when the current through the patient body remains close to 50 μA. In such a case the microcontroller disables the power supply of the measuring boards.
To get optimal signal quality, all recorded signals are originally measured relatively to the properly placed CMS electrode. Potentials of unipolar electrocardiographic leads are then computed in the PC by subtracting the WCT potential from the electrode signals, while bipolar leads are computed as difference signals between corresponding electrodes. Each measuring channel is low-noise (< 0.75 μVRMS) and equipped with a DC-coupled instrumentation amplifier (Analog Devices AD 627) with fixed gain of 40 and a 22-bit Σ-δ A/D converter (Analog Devices AD 7716). Sampling frequency can be selected between 125 and 2000 Hz and provides corresponding effective dynamic resolution between 19 and 16 bits.
The data acquisition system is controlled by a 16-bit CISC Fujitsu microcontroller placed on the motherboard. It scans the data sampled from analogue channels and sends it to the connected host PC. To minimize the capacitive coupling between the USB port of the host PC and the patient terminal, a fibre optic USB extension cable is used.
Disposable Ag-AgCl electrodes with active adapters are used for ECG signals sensing. Each electrode is made in SMD technology and has a thermally compensated amplifier (Analog Devices OP 193). Therefore each electrode has high input and very low output impedance, what effectively reduces disturbing signals, guarantees low noise induced in electrode cables and enables high quality ECG measurement. Disposable electrodes usage eliminates risk of patient infections as well.
The host personal computer of the ProCardio-8 system runs Microsoft Windows® based measuring and data analysis application software containing five basic modules:
- Configuration of the data acquisition system,
- Testing of electrode contacts and signal calibration,
- Measurement of ECG signals,
- Off-line ECG signal processing,
- Computation and analysis of body surface potential maps.
The first three user-friendly programmed modules enable to set all necessary parameters of the system, to test electrode-skin connections and optimize signal processing depending on ECG amplitudes, read the ECG data stream into the host PC, monitor recorded ECG signals on the screen and store recorded measurements. Measured and processed data are stored as GDF files (general data format for biosignals) in selected directory. The fourth module performs off-line ECG signal filtering and baseline correction, enables to select desired time instants in the ECG records and finally the fifth module computes, displays and analyses body surface potential maps, integral maps and desired difference or departure integral maps.
Creating, debugging and consecutive testing of any real-time measuring software is a very difficult and time consuming process, especially in so sensitive field as medical sciences. The classical approach is to develop an application source code in C++ language what certainly takes a large amount of programmer’s time and labour. To simplify and accelerate this process, we decided to use the MATLAB environment and its supporting background also for measurement control and communication with the external hardware. This concept offers the opportunity to utilize all digital signal processing and analysis functions included in the MATLAB for processing of the data stream during the data acquisition and subsequent off-line processing and analysis of measured ECG signals [3].
Cardiac pathology localization method
The location of some cardiac pathology can be found noninvasively by solving the inverse problem of electrocardiology. In our study, possibility to locate one or two ischemic areas with changed repolarization was analysed.
The noninvasive inverse method was based on a dipole model of the cardiac electric generator representing small pathological lesions and realistic model of the geometry and electrical properties of an inhomogeneous human torso [5], [6].
The method evaluates differences between surface potentials recorded in the same patient under conditions without manifestation of ischemia and during ischemia developed during a stress test or by progress of the disease.
Differences within the depolarization - repolarization period can be topologically represented as one difference integral map (DIM) showing the surface distribution of differences between integrals of ECG potentials over the QRST interval recorded in corresponding surface points. Assuming a multiple dipole generator and piecewise homogeneous torso as the volume conductor, boundary element method can be applied for computation of body surface potentials yielding a linear matrix equation.
Computation of the DIM by subtracting the normal integral map from the integral map with manifested ischemia is then equivalent to the computation of an integral map for a difference multiple dipole generator Δs representing only the pathological changes:
(1) where Δp is the vector of computed values in the DIM, A is the transfer matrix representing the torso volume conductor, si and sn are dipolar sources in the normal and ischemic myocardium and Δs represents dipolar generators in ischemic lesions; physically s represents time integrals of dipole moments of current dipoles.
In the inverse solution, a single dipole or a pair of dipoles was searched as the equivalent generator (EG) representing the small lesions. The inverse solution was based on singular value decomposition of a submatrix of the transfer matrix A. The submatrix was composed of columns of the matrix A corresponding to every possible position of an EG located in the predefined points evenly distributed within the modelled ventricular myocardium (N possible dipoles, or N.(N-1)/2 possible dipole pairs).
As the transfer submatrix for each dipole or pair of dipoles is strongly over-determined, unique solution in the sense of the minimum least-squares criterion can be always obtained. The best representative dipole or pair of dipoles with minimal rms difference (DIF) between the measured DIM and the map generated by the inversely estimated equivalent generator was then considered to represent the analysed cardiac pathology.
However, especially for dipole pairs, this criterion usually had no sharp minimum and several dipole pairs gave results with DIF varying very slightly from the minimum. Therefore also results with DIF within 1% difference from the best solution were analysed. To identify the cases, where the inversely computed dipoles really represented 2 lesions, two clusters of dipoles were created from the obtained dipole pairs by applying the modified K-means iterative algorithm for K=2, based on Euclidean distance between the dipoles. Dipole positions of the dipole pair with smallest DIF value were used as the initial positions of the cluster centres. Dipoles from another dipole pairs were then assigned to the cluster with the nearest cluster centre.
Because dipoles from one pair should represent different ischemic lesions, they should belong to different clusters. If both dipoles were assigned to the same cluster, the pair was excluded from the evaluation. At the end of each iteration, new cluster centres were recalculated from assigned dipoles and next iteration was started. If no more changes occurred during the iteration, the algorithm finished dividing dipoles into 2 clusters. The final gravity centre of each cluster was considered as the centre of an identified lesion and the mean dipole moment computed from all dipoles in one cluster was assigned to that lesion. At the end of the iteration process, the DIM was claimed to represent two distinct lesions only if all pairs of the inverse dipoles have their dipoles located in different clusters and the distance between the clusters was big enough.
Patient analysis
To locate the heart repolarization changes, body surface potentials from multiple chest leads were measured using the ProCardio-8 in a group of patients (8 men and 3 women, age 45-69) after myocardial infarction and surface QRST integral maps were computed. In order to calculate equivalent current dipoles representing the regions with changed repolarization, inhomogeneous torso model with lungs and both, realistic and analytical heart model were used. Integral maps before and during the exercise test at load of 75W were computed for each patient and the corresponding EG was considered as the representation of possible local repolarization changes induced due to the stress test and was compared with perfusion images obtained by single photon emission computer tomoghraphy (SPECT). Analysis of the results showed that in 80% of cases the EG representing the QRST DIMs was located in areas identified also by the SPECT, so the method could be a useful tool for localization of ischemic heart lesions [5].
Moreover, information on individual torso structure from MRI, CT or ultrasound systems could significantly increase the quality and accuracy of this advanced inverse diagnostic method for identification of local repolarization changes [6].
Ventricular arrhythmias belong to the main causes of mortality in the western society. Ablation of the ectopic centre, which causes ventricular cardiac rhythm pathology, can significantly improve the stability of the heart function and decrease the number of life threatening arrhythmias. O. Dössel’s group [7] has used anatomical data of the patient and a model based on the cellular automaton principle to demonstrate possibility of ectopic focus localization. Their method showed reliable localization of premature ventricular beat foci with reconstruction error less than 6.1 mm. Therefore we are going to supplement the ProCardio-8 software with new algorithms which will enable to detect ventricular ectopic centres using the Tikhonov regularization method [8].
Conclusion
Analysis of the normal electrophysiology of the heart, cardiac arrhythmia mechanisms origin and ECG genesis specifics showed that using body surface potentials together with patient torso model (using cardiac CT or MRI scans) is possible instrument for cardiac arrhythmia substrate localization. Physical and mathematical aspects of the inverse solution using simplified dipole-based model of cardiac pathologies were experimentally verified and ProCardio-8 mapping system, for computation and evaluation of body surface potential maps was developed. Efficiency of the solution of the inverse problem of electrocardiography and its possibility to locate cardiac pathologies was demonstrated on localization of one or two simultaneous ischemic lesions with changed repolarization properties.
Further software development will include ventricular premature beat foci localization. Application of this method for arrhythmia diagnostics can expand understanding of electrophysiological processes in the heart in different clinical cases.
Acknowledgement
This work has been supported by the research grants No. NT/11532-5 from the Ministry of Health of the Czech Republic IGA, No. SGS11/143/OHK5/2T/17 from Czech Technical University in Prague SGS, No. 2/0210/10 from the VEGA Grant Agency and No. APVV-0513-10 from the Slovak Research and Development Agency.
Olena Punshchykova, Mgr.
Department of Biomedical Technology
Faculty of Biomedical Engineering
Czech Technical University in Prague
nám. Sítná 3105, CZ-272 01 Kladno
E-mail: olena.punshchykova@fbmi.cvut.cz
Phone: +420 777 093 734
Sources
[1] Vardas, P., Auricchio, A., Merino, J.L. The EHRA White Book 2011. The Current Status of Cardiac Electrophysiology in ESC Member Countries. p. 498, www.escardio.org/EHRA.
[2] Karas, S., Hebláková, E., Rosík, V., Tyšler, M. Multichannel high-resolution electrocardiograph developed in Matlab. Technical Computing Prague. 16th Annual Conference Proceedings, Praha, Czech Republik, 2008, p. 55.
[3] Tyšler, M., Rosík, V., Kneppo, P. Multichannel ECG measurement for noninvasive identification of heart regions with changed repolarization. XVIII IMEKO World Congress. Metrology for a Sustainable Development, Rio de Janeiro, Brasil, 2006.
[4] Rosík, V., Karas, S., Hebláková, E., Tyšler, M., Filipová, S. Portable device for high resolution ECG mapping. Measurement science review, 2007, vol. 7, no. 6, p. 57-61.
[5] Tyšler, M., Svehlikova, J., Rosík, V., Karas, S., Hebláková, E., Kneppo, P., Muzik, J., Kania, M., Zaczek R., Kobylecka, M. Model-based method and instrumentation for noninvasive identification of local ischemic lesions in the heart. World Congress on Medical Physics and Biomedical Engineering. IFMBE Proceedings, Munich, Germany, 2009, p.1403-1406.
[6] Svehlikova, J., Lenkova, J., Turzova, M., Tysler, M., Kania, M., Maniewski R. Influence of individual torso geometry on inverse solution to 2 dipoles. J Electrocardiol, 2012, vol. 45, no. 1, p. 7- 12.
[7] Farina, D., Dössel, O. Non-invasive model-based localization of ventricular ectopic centers from multichannel ECG. International Journal of Applied Electromagnetics and Mechanics, 2009, vol. 30, no. 3-4, p. 289-297.
[8] Denisov, A. M., Zakharov, E. V., Kalinin, A. V., Kalinin, V. V. Numerical solution of the inverse electrocardiography problem with the use of the Tikhonov regularization method. Moscow University Computational Mathematics and Cybernetics, 2008, vol. 32, no. 2, p. 61-68.
Labels
BiomedicineArticle was published in
The Clinician and Technology Journal
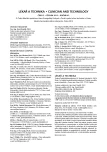
2012 Issue 2
Most read in this issue
- MECHANICAL MODEL OF THE CARDIOVASCULAR SYSTEM: DETERMINATION OF CARDIAC OUTPUT BY DYE DILUTION
- MATLAB AND ITS USE FOR PROCESSING OF THERMOGRAMS
- VENTILATOR CIRCUIT MODEL FOR OPTIMIZATION OF HIGH-FREQUENCY OSCILLATORY VENTILATION
- SETTING EMG STIMULATION PARAMETERS BY MICROCONTROLLER MSP430