WIRELLES PROBE FOR HUMAN BODY BIOSIGNALS
Authors:
Martin Daricek; Martin Jagelka; Lubomir Sladek; Frantisek Horinek; Peter Miklovic
Authors‘ workplace:
Institute of Electronics and Photonics, Slovak University of Technology in Bratislava, Slovakia
Published in:
Lékař a technika - Clinician and Technology No. 2, 2012, 42, 42-45
Category:
Conference YBERC 2012
Overview
This paper describes in detail the multi-purpose device (probe) for wireless biosignal measurement. The proposed system uses two electrodes measurement of the potential and the measured data is sent in real time to user's computer for processing. It is possible to measure simultaneously at several places on the body, while single devices are time synchronized. At this time, designed system can measure two basic biosignals in the human body, EMG and ECG. There are also capabilities for EEG measurements, but it hasn’t been implemented yet.
Keywords:
Wireless biosignal probe, EMG, ECG
Introduction
There is a lot of existing equipment for measuring the biosignals. Most of them are not mobile enough to avoid affecting the measurement, particularly if investigated person is under physical load. This paper describes hardware of wireless bio-probe, which is part of our sensing network, dedicated to capture biosignals from human body using non-invasive application.
In general, EMG and ECG biosignal measurement includes electrical differential potential measurement captured from electrodes applied to skin of human body. There is also possibility of non-contact measurement, based on capacitive coupling, but in this case, we used contact method. EMG and ECG signals could by described as alternating voltage with bandwidth 1 to 300 Hz, with amplitude less than 10 mV. This range is also the strong source of interference, mainly due power line. This is the reason, why most of conventional systems use three electrode connection, where two of them are used for differential potential sensing and the last one serves as potential pre-bias and compensation for common mode interference rejection. We use only two electrodes, because there is no galvanic connection between different devices of the system and also electrodes are places very close to processing circuitry.
Description of device
This design is 4th device version, which was developed during last few years [1], [2], [3]. The entire device consists of several parts, including two main circuit boards (PCB), battery and casing with integrated electrodes (fig. 1). Casing is made from polyurethane and electrodes are made from aluminum coated by evaporated platinum.
Power supply
The power supply is provided designed by integrated single cell Li-Po cell with a capacity of 120 mAh. Both, analog and digital PCBs are supplied from its own LDO and there is also separated ground. This is done due to proper insulation between sensitive analog circuitry and fast switched digital signals on digital PCB. Charging is handled by MCP73831T integrated circuit having a programmable charge current. Device can be charged from USB port or USB compatible wall/car charger. 250 mA charge current ensures full charge cycle in 30 minutes. Current consumption of the device is on average 20 mA, which designates up to 6 hours of working time. Each electronic component used in this device is able to operate from 2.7 V of supply voltage.
Analog circuitry
The analog frontend circuitry is essential for differential sensing of potential on the skin surface. Most signals in the human body are represented by low power differences between two points. We therefore designed a system whose block diagram is shown in Figure 2.
Low power signal, captured by two platinum electrodes, pass through the second order passive low pass filter to INA326, low noise, high precision instrumentation amplifier. The corner frequency of the low pass filter, which helps to suppress ineligible interference, is set to 1 kHz. To ensure correct function of instrumentation amplifier, both electrodes are through high impedance resistor pre-biased to virtual ground voltage level, because there is no third electrode, which usually provides this function.
Due to supply voltage is set to 3 V, reference or virtual ground voltage is set to 1.5 V. Input instrumentation amplifier is supplemented by high pass filter, which is used to eliminate offsets, thermal floating and other DC instabilities. The filter is designed as non-pass filter, which means that output signal does not pass through this filter, to avoid any additional distortion, but it is placed in INA326 reference bias circuit. Instrumentation amplifier gain is 10, which is set by external components.
The next signal processing provides analog to digital converter (ADC) represented by ADS1246, highly integrated and accurate 24-bit ΔΣ ADC. It has a low noise built-in programmable amplifier, internal oscillator and adjustable digital filter with differential input. Programmable amplifier is a low noise and low drift with adjustable amplification in range 1 to 128 in 8 steps. ADC input range is given by/between two reference voltage levels (1 V and 2 V). The frequency modulator can be varied from its value, then the sampling frequency is derived (Table 2). There is also integrated low pass digital FIR filter, whose cut-off frequency is given by actual sample rate, which can be set from 5 to 2000 SPS in 10 steps. For rates lower than 160 SPS filter is automatically configured to additionally suppress 50 Hz component from filtered spectra [4]. Two differential inputs of ADC are connected to instrumentation amplifier output signal and to 1.5 V virtual ground reference voltage.
We used integrated shunt reference IC to produce all three desired reference voltages. Class B of LM4040, provides high stability and accuracy of 2V voltage. All nets using any of reference voltages are high impedance types, so we were allowed to place an resistor divider as 1.5 and 1 V voltage sources.
Digital part
The core of the digital PCB is JN5148, 32-bit RISC microcontroller (MCU, 128 kB RAM, 4 MB FLASH), which includes digital radio transceiver working in ISM band (2.4 GHz) based on IEEE 802.15.4 standard. Standard ZigBee Pro network or Jennet type network can be used to set wireless system [5].
The probe is equipped with 14-bit digital accelerometer, which is used for motion sensing and for power management. There is no mechanical component used for switching the device on and off, therefore if there is any motion noticed, accelerometer will generate digital wake signal for MCU. Device is powered off, if there is no motion for defined time period. Accelerometer communicates with MCU through onboard TWI interface, while SPI interface is used for capturing data from ADC. RGB LED, driven by three PWM channels, is used as state indicator (fig. 4).
Up to 16 probes can be connected in wireless sensor network, which consists from USB dongle device, serves as network coordinator, and described probe devices, which are network slaves. All network devices are synchronized in time [6]. Data flow through USB interface to users PC, used for signal processing, storage, visualization and for measurement parameters set up.
Experimental results
To optimize the sensor smart system were carried out several experiments. This chapter lists only some of the recent experiments, proving system abilities. We used professional biomedical laboratory equipment (AD Instruments PowerLAB) for comparison. Experimental setup was installed (fig. 4) to capture the same EMG/ECG signals simultaneously by two independent sensing systems. While electrodes during AD Instruments ECG measurement were in Einthoven triangle configuration, wireless bio-probe was placed right under left breast with horizontally oriented electrodes. ECG measurement Figures 5 and 6, showing raw data, demonstrate a higher signal quality, greater stability, lower noise and greater suppression of undesirable artifacts in case of our wireless probe system (EMGMED). Moreover there is no need of using conductivity gel. Another great advantage is that it provides a portable monitoring system, so exanimated person is not delimited by wiring.
The second experiment provides brief investigation of dependence between the amplitude of the EMG signal and the force exerted by EMG = f (F) (fig. 6). The results achieved by smart sensor system prove theoretical model, which suggest that the dependence should have a logarithmic shape. Most of the measurements, made by other research groups, result in linear dependence.
Conclusion
Experimental results indicate high level usefulness for medicine and sport researches [7]. In the next version of the device, we will implement possibility of conductive measurements. Also PCB design will be optimized using a custom analog design of the radio antenna and the using physically smaller battery. Waterproof construction will be used, which needs implementation of wireless battery charging method. Using the proposed diagnostic system in practice is also strongly supported through the daily use of smartphones with Android or iOS high computing power and functionality that will be compatible with next version of the sensor system.
Acknowledgement
This work was supported by NanoDesign, Ltd. company and the Slovak Research and Development Agency under the contract No. VMSP-II-0016-09 and by Competence Center for SMART Technologies for Electronics and Informatics Systems and Services, ITMS 26240220072, funded by the Research & Development Operational Programme from the ERDF. Also Slovak Grant Agency supported this work though grant no. 1/1177/12
Martin Daříček, Ph.D.
Institute of Electronics and Photonics
Faculty of Electrical Engineering and Information Technology
Slovak Technical University in Bratislava
Ilkovičova 3, SK-812 19 Bratislava
E-mail: martin.daricek@stuba.sk
Phone: +421 903 744 442
Sources
[1] Rendek, K. - Daříček, M. - Vavrinský, E. - Donoval, M. - Donoval, D.: Biomedical signal amplifier for EMG wireless sensor system. In: ASDAM 2010. The Eighth International Conference on Advanced Semiconductor Devices and Microsystems. Smolenice, 25 - 27 October 2010. - Piscataway : Institute of Electrical and Electronics Engineers, 2010. - ISBN 978-1-4244- 8572-7
[2] Vavrinský, E. - Rendek, K. - Daříček, M. - Donoval, M. - Horínek, F. - Horniak, M. - Donoval, D.: Electrode configuration for EMG measurements. In: ASDAM 2010. The Eighth International Conference on Advanced Semiconductor Devices and Microsystems. Smolenice, 25 - 27 October 2010. - Piscataway : Institute of Electrical and Electronics Engineers, 2010. - ISBN 978-1-4244-8572-7
[3] Vavrinský, E. - Daříček, M. - Donoval, M. - Rendek, K. - Horínek, F. - Horniak, M. - Donoval, D.: Design of EMG Wireless Sensor System. In: Applied Electronics 2011 : International Conference on Applied Electronics. Pilsen, 7-8 September 2011. - Pilsen : University of West Bohemia, 2011. - ISBN 978-80- 7043-987-6. - S. 401-404
[4] ADS1246/47/48 datasheet, http://www.ti.com/lit/ds/symlink/ads1246.pdf
[5] JN5148-001-Myy datasheet, http://www.jennic.com/files/product_briefs/JN-DS-JN5148MO- 1v4.pdf
[6] Daříček, M., „Synchronization Methods for Wireless Biosensing Network“, Proceeding of 22nd International Conference Radioelektronika 2012, Brno, pp. 157-160, ISBN 978-80-214- 4468-3
[7] Vavrinský, E., Praktický monitoring biopotenciálov pomocou nízkošumového bezdrôtového senzora, Transfer, 1, 2012, str. 16-17, ISSN 1337-9747
Labels
BiomedicineArticle was published in
The Clinician and Technology Journal
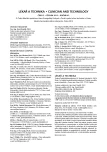
2012 Issue 2
Most read in this issue
- MECHANICAL MODEL OF THE CARDIOVASCULAR SYSTEM: DETERMINATION OF CARDIAC OUTPUT BY DYE DILUTION
- MATLAB AND ITS USE FOR PROCESSING OF THERMOGRAMS
- VENTILATOR CIRCUIT MODEL FOR OPTIMIZATION OF HIGH-FREQUENCY OSCILLATORY VENTILATION
- SETTING EMG STIMULATION PARAMETERS BY MICROCONTROLLER MSP430