CRISPR-Cas9 as a Tool in Cancer Therapy
Authors:
P. Zatloukalová; R. Krejčíř; D. Valík; B. Vojtěšek
Authors‘ workplace:
Regionální centrum aplikované molekulární onkologie, Masarykův onkologický ústav, Brno
Published in:
Klin Onkol 2019; 32(Supplementum 3): 13-18
Category:
Review
doi:
https://doi.org/10.14735/amko20193S
Overview
Background: Genome editing using CRISPR-Cas9 has become one of the basic methods of biological research over a short period of time. This recently discovered system of adaptive immunity of bacteria has been adapted to the needs of science and has become a valuable tool for DNA manipulation. Its simplicity and reliability have contributed to widespread use of the method. Genome editing refers to targeted modifications of genomic DNA with single base pair accuracy. CRISPR-Cas9 differs significantly from previous technologies in the simplicity of directing the enzyme to the target sequence. In the field of cancer research, CRISPR-Cas9 has enabled the development of a number of models for the study of carcinogenesis and drug testing. From a therapeutic point of view, CRISPR-Cas9 has been applied in the field of immunotherapy, especially in ex vivo genetic modifications of the T-cells of patients.
Aim: Currently, several clinical trials are trying to verify the therapeutic potential of CRISPR-Cas9. Based on these studies, we have summarised the strategies used in the preparation of therapeutic tools useful in cancer therapy.
Conclusion: CRISPR-Cas9 appears to be crucial in basic research, particularly in the study of the function of individual genes involved in carcinogenesis. However, it will still be necessary to optimise the efficacy, safety and specificity of CRISPR-Cas9 before it is used in clinical practice.
Keywords:
CRISPR-Cas9 – immunotherapy – clinical trial
Sources
1. Cong L, Ran FA, Cox D et al. Multiplex genome engineering using CRISPR/ Cas systems. Science 2013; 339(6121): 819– 823. doi: 10.1126/ science.1231143.
2. Lander ES. The Heroes of CRISPR. Cell 2016; 164(1– 2): 18– 28. doi: 10.1016/ j.cell.2015.12.041.
3. Gupta RM, Musunuru K. Expanding the genetic editing tool kit: ZFNs, TALENs, and CRISPR-Cas9. J Clin Invest 2014; 124(10): 4154– 4161. doi: 10.1172/ JCI72992.
4. Adli M. The CRISPR tool kit for genome editing and beyond. Nat Commun 2018; 9(1): 1911. doi: 10.1038/ s41467-018-04252-2.
5. Chen B, Gilbert LA, Cimini BA et al. Dynamic imaging of genomic loci in living human cells by an optimized CRISPR/ Cas system. Cell 2013; 155(7): 1479– 1491. doi: 10.1016/ j.cell.2013.12.001.
6. Shachaf CM, Kopelman AM, Arvanitis C et al. MYC inactivation uncovers pluripotent differentiation and tumour dormancy in hepatocellular cancer. Nature 2004; 431(7012): 1112– 1117. doi: 10.1038/ nature03043.
7. Rouet P, Smih F, Jasin M. Introduction of double-strand breaks into the genome of mouse cells by expression of a rare-cutting endonuclease. Mol Cell Biol 1994; 14(12): 8096– 8106. doi: 10.1128/ mcb.14.12.8096.
8. Klann TS, Black JB, Chellappan M et al. CRISPR-Cas9 epigenome editing enables high-throughput screening for functional regulatory elements in the human genome. Nat Biotechnol 2017; 35(6): 561– 568. doi: 10.1038/ nbt.3853.
9. Zatloukalova P, Pjechova M, Babcanova S et al. The role of PD-1/ PD-L1 signaling pathway in antitumor immune response. Klin Onkol 2016; 29 (Suppl 4): 72– 77. doi: 10.14735/ amko20164S72.
10. Barber DL, Wherry EJ, Masopust D et al. Restoring function in exhausted CD8 T cells during chronic viral infection. Nature 2006; 439(7077): 682– 687. doi: 10.1038/ nature04444.
11. Biffi A, Bartolomae CC, Cesana D et al. Lentiviral vector common integration sites in preclinical models and a clinical trial reflect a benign integration bias and not oncogenic selection. Blood 2011; 117(20): 5332– 5339. doi: 10.1182/ blood-2010-09-306761.
12. Park JH, Geyer MB, Brentjens RJ. CD19-targeted CAR T-cell therapeutics for hematologic malignancies: interpreting clinical outcomes to date. Blood 2016; 127(26): 3312– 3320. doi: 10.1182/ blood-2016-02-629063.
13. Xia AL, Wang XC, Lu YJ et al. Chimeric-antigen receptor T (CAR-T) cell therapy for solid tumors: challenges and opportunities. Oncotarget 2017; 8(52): 90521– 90531. doi: 10.18632/ oncotarget.19361.
14. Eshhar Z, Waks T, Gross G et al. Specific activation and targeting of cytotoxic lymphocytes through chimeric single chains consisting of antibody-binding domains and the gamma or zeta subunits of the immunoglobulin and T-cell receptors. Proc Natl Acad Sci USA 1993; 90(2): 720– 724. doi: 10.1073/ pnas.90.2.720.
15. Eyquem J, Mansilla-Soto J, Giavridis T et al. Targeting a CAR to the TRAC locus with CRISPR/ Cas9 enhances tumour rejection. Nature 2017; 543(7643): 113– 117. doi: 10.1038/ nature21405.
16. Tang Z, Qian M, Ho M. The role of mesothelin in tumor progression and targeted therapy. Anticancer Agents Med Chem 2013; 13(2): 276– 280.
17. Ren J, Zhang X, Liu X et al. A versatile system for rapid multiplex genome-edited CAR T cell generation. Oncotarget 2017; 8(10): 17002– 17011. doi: 10.18632/ oncotarget.15218.
Labels
Paediatric clinical oncology Surgery Clinical oncologyArticle was published in
Clinical Oncology
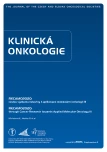
2019 Issue Supplementum 3
Most read in this issue
- Protein Ubiquitination Research in Oncology
- CRISPR-Cas9 as a Tool in Cancer Therapy
- Glycosylation as an Important Regulator of Antibody Function
- Uncommon EGFR Mutations in Non-Small Cell Lung Cancer and Their Impact on the Treatment