ATP-sensitive potassium channels: key players in pathophysiology of many diseases
Authors:
Kateřina Bláhová; Markéta Bébarová
Authors‘ workplace:
Fyziologický ústav LF MU v Brně
Published in:
Čas. Lék. čes. 2021; 160: 71-80
Category:
Review Article
Overview
ATP-sensitive potassium channels have been an intensively studied type of protein complexes incorporated in the cell membrane for several decades. Their unique function makes them special, as they create a connection between the metabolic state and membrane voltage of the cell. This position of a bridge involved in many cellular cascades allow them to participate in various processes at often surprising positions in nearly all organ systems of the body, from the pancreas, heart muscle or retina, to the central nervous system. This review summarizes the most important roles of ATP-sensitive potassium channels focusing on their possible clinical use within particular organ systems.
Keywords:
ATP-sensitive potassium channels – mitochondria – diabetes – Vasodilation – ischemic preconditioning – Arrhythmia – Cantú syndrome – leptin – Parkinsonʻs disease – Alzheimerʻs disease
Sources
- Nalgirkar V. K+ channels: ATP-sensitive and O2-sensitive. 2019 Nov. Dostupné na: www.youtube.com/watch?v=s1Lxy_9Vma0
- Noma A. ATP-regulated K+ channels in cardiac muscle. Nature 1983; 305(5930): 147–148.
- Huang Y, Hu D, Huang C et al. Genetic discovery of ATP-sensitive K+ channels in cardiovascular diseases. Circ Arrhythm Electrophysiol 2019; 12(5): e007322.
- Hibino H, Inanobe A, Furutani K et al. Inwardly rectifying potassium channels: their structure, function, and physiological roles. Physiol Rev 2010; 90(1): 291–366.
- Miki T, Suzuki M, Shibasaki T et al. Mouse model of Prinzmetal angina by disruption of the inward rectifier Kir6.1. Nat Med 2002; 8(5): 466–472.
- Aziz Q, Thomas AM, Gomes J et al. The ATP-sensitive potassium channel subunit, Kir6.1, in vascular smooth muscle plays a major role in blood pressure control. Hypertension 2014; 64(3): 523–529.
- Li A, Knutsen RH, Zhang H et al. Hypotension due to Kir6.1 gain‐of‐function in vascular smooth muscle. J Am Heart Assoc Cardiovasc Cerebrovasc Dis 2013; 2(4).
- Fatehi M, Carter CC, Youssef N et al. The mechano-sensitivity of cardiac ATP-sensitive potassium channels is mediated by intrinsic MgATPase activity. J Mol Cell Cardiol 2017; 108: 34–41.
- Paggio A, Checchetto V, Campo A et al. Identification of an ATP-sensitive potassium channel in mitochondria. Nature 2019; 572(7771): 609–613.
- Minatoguchi S, Uno Y, Kariya T et al. Cross-talk among noradrenaline, adenosine and protein kinase C in the mechanisms of ischemic preconditioning in rabbits. J Cardiovasc Pharmacol 2003; 41 (Suppl. 1): 39–47.
- Akao M, Ohler A, O’Rourke B et al. Mitochondrial ATP-sensitive potassium channels inhibit apoptosis induced by oxidative stress in cardiac cells. Circ Res 2001; 88(12): 1267–1275.
- Teshima Y, Akao M, Li RA et al. Mitochondrial ATP-sensitive potassium channel activation protects cerebellar granule neurons from apoptosis induced by oxidative stress. Stroke 2003; 34(7): 1796–1802.
- Walewska A, Szewczyk A, Koprowski P. Gas signaling molecules and mitochondrial potassium channels. Int J Mol Sci 2018; 19(10): 3227.
- Tang G, Wu L, Liang W et al. Direct stimulation of KATP channels by exogenous and endogenous hydrogen sulfide in vascular smooth muscle cells. Mol Pharmacol 2005; 68(6): 1757–1764.
- Suzuki M, Li RA, Miki T et al. Functional roles of cardiac and vascular ATP-sensitive potassium channels clarified by Kir6.2-knockout mice. Circ Res 2001; 88(6): 570–577.
- Bohnen MS, Ma L, Zhu N et al. Loss-of-function ABCC8 mutations in pulmonary arterial hypertension. Circ Genomic Precis Med 2018; 11(10): e002087.
- Elrod JW, Harrell M, Flagg TP et al. Role of sulfonylurea receptor type 1 subunits of ATP-sensitive potassium channels in myocardial ischemia/reperfusion injury. Circulation 2008; 117(11): 1405–1413.
- Flagg TP, Patton B, Masia R et al. Arrhythmia susceptibility and premature death in transgenic mice overexpressing both SUR1 and Kir6.2[ΔN30,K185Q] in the heart. Am J Physiol-Heart Circ Physiol 2007; 293(1): H836–H845.
- McClenaghan C, Hanson A, Sala-Rabanal M et al. Cantu syndrome–associated SUR2 (ABCC9) mutations in distinct structural domains result in KATP channel gain-of-function by differential mechanisms. J Biol Chem 2018; 293(6): 2041–2052.
- Theis NJ, Calvert T, McIntyre P et al. Cantu syndrome and hypopituitarism: implications for endocrine monitoring. Endocrinol Diabetes Amp Metab Case Rep 2019.
- Cho JH, Kang E, Lee BH et al. DEND syndrome with heterozygous KCNJ11 mutation successfully treated with sulfonylurea. J Korean Med Sci 2017; 32(6): 1042.
- Lebl J, Roženková K, Průhová Š. Congenital hyperinsulinism: loss of B-cell self-control. Vnitř Lékařství. 2016; 62(11): 4S72–4S76.
- Amaratunga SA, Hussein Tayeb T, Roženková K et al. Congenital hyperinsulinism caused by novel homozygous KATP channel gene variants may be linked to unexplained neonatal deaths among Kurdish consanguineous families. Horm Res Paediatr 2020; 93(1): 58–65.
- Roženková K, Malíková J, Nessa A et al. High incidence of heterozygous ABCC8 and HNF1A mutations in Czech patients with congenital hyperinsulinism. J Clin Endocrinol Metab 2015; 100(12): E1540–E1549.
- Ye P, Zhu YR, Gu Y et al. Functional protection against cardiac diseases depends on ATP-sensitive potassium channels. J Cell Mol Med 2018; 22(12): 5801–5806.
- Zhang H, Flagg TP, Nichols CG. Cardiac sarcolemmal KATP channels: latest twists in a questing tale! J Mol Cell Cardiol 2010; 48(1): 71–75.
- Nozadze E, Arutinova N, Tsakadze L et al. Molecular mechanism of Mg-ATPase activity. J Membr Biol 2015; 248(2): 295–300.
- Pertiwi KR, Hillman R, Scott CA et al. Ischemia reperfusion injury produces, and ischemic preconditioning prevents, rat cardiac fibroblast differentiation: role of KATP channels. J Cardiovasc Dev Dis 2019; 6(2): 22.
- Zhong YS, Wang J, Liu WM et al. Potassium ion channels in retinal ganglion cells (Review). Mol Med Rep 2013; 8(2): 311–319.
- Pinton P, Giorgi C, Siviero R et al. Calcium and apoptosis: ER-mitochondria Ca2+ transfer in the control of apoptosis. Oncogene 2008; 27(50): 6407–6418.
- Ošťádalová I. Ischemic preconditioning in chronically hypoxic neonatal rat heart. Pediatr Res 2002; 52(4): 561–567.
- Doul J, Miková D, Rašková M et al. Possible role of mitochondrial K-ATP channel and nitric oxide in protection of the neonatal rat heart. Mol Cell Biochem 2019; 450(1): 35–42.
- Borges JP, da Silva Verdoon K. Cardiac ischemia/reperfusion injury: the beneficial effects of exercise. In: Exercise for cardiovascular disease prevention and treatment. Advances in Experimental Medicine and Biology. Springer, Singapur, 2017: 155–179.
- Riess ML, Stowe DF, Warltier DC. Cardiac pharmacological preconditioning with volatile anesthetics: from bench to bedside? Am J Physiol Heart Circ Physiol 2004; 286(5): H1603–H1607.
- Kavazis AN. Exercise preconditioning of the myocardium. Sports Med 2009; 39(11): 923–935.
- Przyklenk K, Bauer B, Ovize M et al. Regional ischemic “preconditioning” protects remote virgin myocardium from subsequent sustained coronary occlusion. Circulation 1993; 87(3): 893–899.
- Hausenloy DJ, Yellon DM. The second window of preconditioning (SWOP): where are we now? Cardiovasc Drugs Ther 2010; 24(3): 235–254.
- Brayden JE. Functional roles of KATP channels in vascular smooth muscle. Clin Exp Pharmacol Physiol 2002; 29(4): 312–316.
- Centeno JM, López-Morales MA, Aliena-Valero A et al. Potassium channels contribute to the increased sensitivity of the rabbit carotid artery to hydrogen sulfide in diabetes. Eur J Pharmacol 2019; 853: 33–40.
- Denizalti M, Bozkurt TE, Akpulat U et al. The vasorelaxant effect of hydrogen sulfide is enhanced in streptozotocin-induced diabetic rats. Naunyn Schmiedebergs Arch Pharmacol 2011; 383(5): 509–517.
- Emfinger CH, Welscher A, Yan Z et al. Expression and function of ATP-dependent potassium channels in zebrafish islet β-cells. R Soc Open Sci 4(2):160808.
- Bell GI, Kayano T, Buse JB et al. Molecular biology of mammalian glucose transporters. Diabetes Care 1990; 13(3): 198–208.
- Cochrane V, Shyng SL. Leptin-induced trafficking of KATP channels: a mechanism to regulate pancreatic β-cell excitability and insulin secretion. Int J Mol Sci 2019; 20(11): 2660.
- Kieffer TJ, Habener JF. The adipoinsular axis: effects of leptin on pancreatic β-cells. Am J Physiol-Endocrinol Metab 2000; 278(1): E1–E14.
- Dubuc PU. The development of obesity, hyperinsulinemia, and hyperglycemia in ob/ob mice. Metab Clin Exp 1976; 25(12): 1567–1574.
- Pelleymounter MA, Cullen MJ, Baker MB et al. Effects of the obese gene product on body weight regulation in ob/ob mice. Science 1995; 269(5223): 540–543.
- Marquard J, Otter S, Welters A et al. Characterization of pancreatic NMDA receptors as possible drug targets for diabetes treatment. Nat Med 2015; 21(4): 363–372.
- Obolensky A, Berenshtein E, Konijn AM et al. Ischemic preconditioning of the rat retina: protective role of ferritin. Free Radic Biol Med 2008; 44(7): 1286–1294.
- Ettaiche M. ATP-sensitive potassium channels (KATP) in retina: a key role for delayed ischemic tolerance. Brain Res 2001; 890(1): 118–129.
- Roy Chowdhury U, Dosa PI, Fautsch MP. ATP-sensitive potassium channel openers: a new class of ocular hypotensive agents. Exp Eye Res 2017; 158: 85–93.
- Varcholová D. Latanoprostum. Remedia 2004; 14(5): 406–413.
- Peng K, Hu J, Xiao J et al. Mitochondrial ATP-sensitive potassium channel regulates mitochondrial dynamics to participate in neurodegeneration of Parkinson’s disease. Biochim Biophys Acta BBA – Mol Basis Dis 2018; 1864(4, Part A): 1086–1103.
- Peng K, Yang L, Wang J et al. The interaction of mitochondrial biogenesis and fission/fusion mediated by PGC-1α regulates rotenone-induced dopaminergic neurotoxicity. Mol Neurobiol 2017; 54(5): 3783–3797.
- Wang J, Li Z, Feng M et al. Opening of astrocytic mitochondrial ATP-sensitive potassium channels upregulates electrical coupling between hippocampal astrocytes in rat brain slices. PLoS One 2013; 8(2): e56605.
- Hu ZL, Sun T, Lu M. Kir6.1/K-ATP channel on astrocytes protects against dopaminergic neurodegeneration in the MPTP mouse model of Parkinson’s disease via promoting mitophagy. Brain Behav Immun 2019; 81: 509–522.
- Moriguchi S, Ishizuka T, Yabuki Y. Blockade of the K ATP channel Kir6.2 by memantine represents a novel mechanism relevant to Alzheimer’s disease therapy. Mol Psychiatry 2016; 23: 211–221.
- Esmaeili MH, Bahari B, Salari AA. ATP-sensitive potassium-channel inhibitor glibenclamide attenuates HPA axis hyperactivity, depression- and anxiety-related symptoms in a rat model of Alzheimer’s disease. Brain Res Bull 2018; 2018(137): 265–276.
- Son Y, Kim K, Cho HR. Sildenafil protects neuronal cells from mitochondrial toxicity induced by β-amyloid peptide via ATP-sensitive K+ channels. Biochem Biophys Reasearch Commun 2018; 500(2): 504–510.
Labels
Addictology Allergology and clinical immunology Angiology Audiology Clinical biochemistry Dermatology & STDs Paediatric gastroenterology Paediatric surgery Paediatric cardiology Paediatric neurology Paediatric ENT Paediatric psychiatry Paediatric rheumatology Diabetology Pharmacy Vascular surgery Pain management Dental HygienistArticle was published in
Journal of Czech Physicians
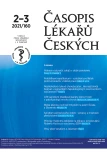
2021 Issue 2-3
Most read in this issue
- COVID-19 antibody testing: examination of the antibody response to coronavirus in common practice
- Computer vision syndrome – symptoms and prevention
- The risk of insect-borne diseases transmission in the Czech Republic and Slovakia
- Non-alcoholic fatty liver disease – how to effectively fight the nowadays most common liver disease?