Detection of cell death markers as a tool for bacterial antimicrobial susceptibility testing
Authors:
P. Mlynárčik; M. Kolář
Authors‘ workplace:
Ústav mikrobiologie, Lékařská fakulta Univerzity Palackého v Olomouci
Published in:
Epidemiol. Mikrobiol. Imunol. 65, 2016, č. 2, s. 85-91
Category:
Review Article
Overview
Antimicrobial resistance among nosocomial pathogens has emerged as one of the most important health care problems in the new millennium. In this review, we present new methods for bacterial antimicrobial susceptibility testing, based on the detection of antibiotic-mediated cell death markers that could provide valuable alternatives to existing phenotypic approaches in the very near future.
KEYWORDS:
cell death – resistance – antimicrobials – Pseudomonas – real-time PCR
Sources
1. Choi M-J, Ko KS. Mutant prevention concentrations of colistin for Acinetobacter baumannii, Pseudomonas aeruginosa and Klebsiella pneumoniae clinical isolates. J Antimicrob Chemother, 2014;69(1):275–277.
2. Canton R, Akova M, Carmeli Y, et al. Rapid evolution and spread of carbapenemases among Enterobacteriaceae in Europe. Clin Microbiol Infect, 2012;18(5):413–431.
3. Cornaglia G, Akova M, Amicosante G, et al. Metallo-beta-lactamases as emerging resistance determinants in Gram-negative pathogens: open issues. Int J Antimicrob Agents, 2007;29(4):380–388.
4. Bou G, Martinez-Beltran J. Cloning, nucleotide sequencing, and analysis of the gene encoding an AmpC beta-lactamase in Acinetobacter baumannii. Antimicrob Agents Chemother, 2000;44(2):428–432.
5. Endimiani A, Luzzaro F, Migliavacca R, et al. Spread in an Italian hospital of a clonal Acinetobacter baumannii strain producing the TEM-92 extended-spectrum beta-lactamase. Antimicrob Agents Chemother, 2007;51(6):2211–2214.
6. Muller C, Plesiat P, Jeannot K. A two-component regulatory system interconnects resistance to polymyxins, aminoglycosides, fluoroquinolones, and beta-lactams in Pseudomonas aeruginosa. Antimicrob Agents Chemother, 2011;55(3):1211–1221.
7. Wang M, Borris L, Aarestrup FM, et al. Identification of a Pseudomonas aeruginosa co-producing NDM-1, VIM-5 and VIM-6 metallo-beta-lactamases in Denmark using whole-genome sequencing. Int J Antimicrob Agents, 2015;45(3):324–325.
8. Cernohorska L, Slavikova P. Antibiotic resistance and biofilm formation in Pseudomonas aeruginosa strains isolated from patients with urinary tract infections. Epidemiol Mikrobiol Imunol, 2009;58(4):154–157.
9. Moker N, Dean CR, Tao J. Pseudomonas aeruginosa increases formation of multidrug-tolerant persister cells in response to quorum-sensing signaling molecules. J Bacteriol, 2010;192(7):1946–1955.
10. Overhage J, Bains M, Brazas MD, et al. Swarming of Pseudomonas aeruginosa is a complex adaptation leading to increased production of virulence factors and antibiotic resistance. J Bacteriol, 2008;190(8):2671–2679.
11. Khakimova M, Ahlgren HG, Harrison JJ, et al. The stringent response controls catalases in Pseudomonas aeruginosa and is required for hydrogen peroxide and antibiotic tolerance. J Bacteriol, 2013;195(9):2011–2020.
12. Hrabak J, Walkova R, Studentova V, et al. Carbapenemase activity detection by matrix-assisted laser desorption ionization-time of flight mass spectrometry. J Clin Microbiol, 2011; 49(9):3222–3227.
13. Mlynarcik P, Kolar M, Roderova M. New technologies for antibiotic susceptibility testing of bacteria. Klin Mikrobiol Infekc Lek, 2014;20(3):72–78.
14. Kerr JF, Wyllie AH, Currie AR. Apoptosis: a basic biological phenomenon with wide-ranging implications in tissue kinetics. Br J Cancer, 1972;26(4):239–257.
15. Dwyer DJ, Camacho DM, Kohanski MA, et al. Antibiotic-induced bacterial cell death exhibits physiological and biochemical hallmarks of apoptosis. Mol Cell, 2012;46(5):561–572.
16. Drlica K, Malik M, Kerns RJ, et al. Quinolone-mediated bacterial death. Antimicrob Agents Chemother, 2008;52(2):385–392.
17. Floss HG, Yu T-W. Rifamycin-mode of action, resistance, and biosynthesis. Chem Rev, 2005;105(2):621–632.
18. Tomasz A. The mechanism of the irreversible antimicrobial effects of penicillins: how the beta-lactam antibiotics kill and lyse bacteria. Annu Rev Microbiol, 1979;33:113–137.
19. Vakulenko SB, Mobashery S. Versatility of aminoglycosides and prospects for their future. Clin Microbiol Rev, 2003;16(3):430–450.
20. Dwyer DJ, Kohanski MA, Collins JJ. Role of reactive oxygen species in antibiotic action and resistance. Curr Opin Microbiol, 2009;12(5):482–489.
21. Kohanski MA, Dwyer DJ, Hayete B, et al. A common mechanism of cellular death induced by bactericidal antibiotics. Cell, 2007;130(5):797–810.
22. Dixon SJ, Stockwell BR. The role of iron and reactive oxygen species in cell death. Nat Chem Biol, 2014;10(1):9–17.
23. Kohanski MA, Dwyer DJ, Wierzbowski J, et al. Mistranslation of membrane proteins and two-component system activation trigger antibiotic-mediated cell death. Cell, 2008;135(4):679–690.
24. Keren I, Wu Y, Inocencio J, et al. Killing by bactericidal antibiotics does not depend on reactive oxygen species. Science, 2013;339(6124):1213–1216.
25. Allison KR, Brynildsen MP, Collins JJ. Metabolite-enabled eradication of bacterial persisters by aminoglycosides. Nature, 2011;473(7346):216–220.
26. Dwyer DJ, Belenky PA, Yang JH, et al. Antibiotics induce redox-related physiological alterations as part of their lethality. Proc Natl Acad Sci USA, 2014;111(20):E2100–E2109.
27. Breidenstein EB, Khaira BK, Wiegand I, et al. Complex ciprofloxacin resistome revealed by screening a Pseudomonas aeruginosa mutant library for altered susceptibility. Antimicrob Agents Chemother, 2008;52(12):4486–4491.
28. Nguyen D, Joshi-Datar A, Lepine F, et al. Active starvation responses mediate antibiotic tolerance in biofilms and nutrient-limited bacteria. Science, 2011;334(6058):982–986.
29. Butala M, Zgur-Bertok D, Busby SJW. The bacterial LexA transcriptional repressor. Cell Mol Life Sci, 2009;66(1):82–93.
30. Erental A, Sharon I, Engelberg-Kulka H. Two programmed cell death systems in Escherichia coli: an apoptotic-like death is inhibited by the mazEF-mediated death pathway. PLoS Biol, 2012;10(3):e1001281.
31. Kolodkin-Gal I, Engelberg-Kulka H. Induction of Escherichia coli chromosomal mazEF by stressful conditions causes an irreversible loss of viability. J Bacteriol, 2006;188(9):3420–3423.
32. Belitsky M, Avshalom H, Erental A, et al. The Escherichia coli extracellular death factor EDF induces the endoribonucleolytic activities of the toxins MazF and ChpBK. Mol Cell, 2011;41(6):625–635.
33. Pandey DP, Gerdes K. Toxin-antitoxin loci are highly abundant in free-living but lost from host-associated prokaryotes. Nucleic Acids Res, 2005;33(3):966–976.
34. Yeom J, Imlay JA, Park W. Iron homeostasis affects antibiotic-mediated cell death in Pseudomonas species. J Biol Chem, 2010;285(29):22689–22695.
35. Aizenman E, Engelberg-Kulka H, Glaser G. An Escherichia coli chromosomal "addiction module" regulated by guanosine [corrected] 3',5'-bispyrophosphate: a model for programmed bacterial cell death. Proc Natl Acad Sci USA, 1996;93(12):6059–6063.
36. Pepper ED, Farrell MJ, Nord G, et al. Antiglycation effects of carnosine and other compounds on the long-term survival of Escherichia coli. Appl Environ Microbiol, 2010; 76(24):7925–7930.
37. Goltermann L, Good L, Bentin T. Chaperonins fight aminoglycoside-induced protein misfolding and promote short-term tolerance in Escherichia coli. J Biol Chem, 2013;288(15): 10483–10489.
38. Carmona-Gutierrez D, Kroemer G, Madeo F. When death was young: an ancestral apoptotic network in bacteria. Mol Cell, 2012;46(5):552–554.
39. Martin-Pena R, Dominguez-Herrera J, Pachon J, et al. Rapid detection of antibiotic resistance in Acinetobacter baumannii using quantitative real-time PCR. J Antimicrob Chemother, 2013;68(7):1572–1575.
40. van Belkum A, Dunne WM, Jr. Next-generation antimicrobial susceptibility testing. J Clin Microbiol, 2013;51(7):2018–2024.
41. Hood MI, Becker KW, Roux CM, et al. genetic determinants of intrinsic colistin tolerance in Acinetobacter baumannii. Infect Immun, 2013;81(2):542–551.
42. Henry R, Vithanage N, Harrison P, et al. Colistin-resistant, lipopolysaccharide-deficient Acinetobacter baumannii responds to lipopolysaccharide loss through increased expression of genes involved in the synthesis and transport of lipoproteins, phospholipids, and poly-beta-1,6-N-acetylglucosamine. Antimicrob Agents Chemother, 2012;56(1):59–69.
Labels
Hygiene and epidemiology Medical virology Clinical microbiologyArticle was published in
Epidemiology, Microbiology, Immunology
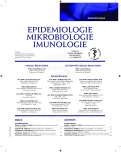
2016 Issue 2
Most read in this issue
- A report of 10 cases of familial Creutzfeld-Jakob disease
- Novel approaches to control the rise in pertussis cases
-
The occurrence of Ixodes ricinus ticks and important tick-borne pathogens in areas with high tick-borne encephalitis prevalence in different altitudinal levels of the Czech Republic
Part I. Ixodes ricinus ticks and tick-borne encephalitis virus - The effect of oxygen on endotoxin production in bacteria of the Bacteroides fragilis group isolated from patients with colorectal carcinoma