Chromothripsis – Extensive Chromosomal Rearrangements and Their Significance in Cancer
Authors:
K. Závacká 1; K. Plevová 1,2; M. Jarošová 1; Š. Pospíšilová 1,2
Authors‘ workplace:
Centrum molekulární bio logie a genové terapie, Interní hematologická a onkologická klinika LF MU a FN Brno
1; Centrum molekulární medicíny, CEITEC – Středoevropský technologický institut, Masarykova univerzita, Brno
2
Published in:
Klin Onkol 2019; 32(2): 101-108
Category:
Review
doi:
https://doi.org/10.14735/amko2019101
Overview
Background:
Chromosome rearrangements play an important role in cancer pathophysiology. Recently, chromothripsis has been proposed among the mechanisms leading to their formation. Chromothripsis leads to fragmentation of chromosomes and their reconstitution with tens to hundreds of rearrangements clustered in small genome regions. In contrast to the traditional concept of malignant transformation, abnormalities caused by chromothripsis are not accumulated gradually but arise during a single event. The resulting structural variants are extensive and often cause oncogene activation or tumor suppressor inactivation. Chromothripsis is associated with many tumor types, especially with brain and bone tumors. Besides that, it has been described also in congenital disorders. The exact mechanism of chromothripsis origin has not been clarified yet; however, several hypotheses have been prosed, among which DNA damage in micronucleus seems to be most likely. Similarly, an impact of chromothripsis on cellular processes has not been fully understood, yet its association with unfavorable prognosis has been observed.
Purpose:
The purpose of this article is to summarize the current knowledge about chromothripsis and to present gathered pieces of information in a structured way. We focused on describing the basic features of chromothripsis, potential mechanisms of its origin, its impact on cellular processes and providing an overview of diseases where chromothripsis has been noted, with particular attention to cancer. Finally, we suggest a potential use of current knowledge about chromothripsis in the optimization of personalized treatment.
Supported by Ministry of Health of the Czech Republic, grant no. 15-31834A. All rights reserved.
The authors declare they have no potential conflicts of interest concerning drugs, products, or services used in the study.
The Editorial Board declares that the manuscript met the ICMJE recommendation for biomedical papers.
Submitted: 31. 12. 2018
Accepted: 19. 3. 2019
Keywords:
chromothripsis – Chromosomes – DNA – Mutation – genomic structural variation – DNA end-joining repair
Sources
1. Stephens PJ, Greenman CD, Fu B et al. Massive genomic rearrangement acquired in a single catastrophic event during cancer development. Cell 2011; 144 (1): 27–40. doi: 10.1016/j.cell.2010.11.055.
2. Kloosterman WP, Koster J, Molenaar JJ. Prevalence and clinical implications of chromothripsis in cancer genomes. Curr Opin Oncol 2014; 26 (1): 64–72. doi: 10.1097/CCO.0000000000000038.
3. Kloosterman WP, Guryev V, van Roosmalen M et al. Chromothripsis as a mechanism driving complex de novo structural rearrangements in the germline. Hum Mol Genet 2011; 20 (10): 1916–1924. doi: 10.1093/hmg/ ddr073.
4. de Pagter MS, van Roosmalen MJ, Baas AF et al. Chromothripsis in healthy individuals affects multiple protein-coding genes and can result in severe congenital abnormalities in offspring. Am J Hum Genet 2015; 96 (4): 651–656. doi: 10.1016/j.ajhg.2015.02.005.
5. Rausch T, Jones DT, Zapatka M et al. Genome sequencing of pediatric medulloblastoma links catastrophic DNA rearrangements with TP53 mutations. Cell 2012; 148 (1–2): 59–71. doi: 10.1016/j.cell.2011.12.013.
6. Holland AJ, Cleveland DW. Chromoanagenesis and cancer: mechanisms and consequences of localized, complex chromosomal rearrangements. Nat Med 2012; 18 (11): 1630–1638. doi: 10.1038/nm.2988.
7. Crasta K, Ganem NJ, Dagher R et al. DNA breaks and chromosome pulverization from errors in mitosis. Nature 2012; 482 (7383): 53–58. doi: 10.1038/nature10802.
8. Liu S, Kwon M, Mannino M et al. Nuclear envelope assembly defects link mitotic errors to chromothripsis. Nature 2018; 561 (7724): 551–555. doi: 10.1038/s41586-018-0534-z.
9. Huang Y, Jiang L, Yi Q et al. Lagging chromosomes entrapped in micronuclei are not “lost” by cells. Cell Res 2012; 22 (5): 932–935. doi: 10.1038/cr.2012.26.
10. Artandi SE, Chang S, Lee SL et al. Telomere dysfunction promotes non-reciprocal translocations and epithelial cancers in mice. Nature 2000; 406 (6796): 641–645.
11. Shim G, Ricoul M, Hempel WM et al. Crosstalk between telomere maintenance and radiation effects: a key player in the process of radiation-induced carcinogenesis. Mutat Res Rev Mutat Res 2014; S1383–S5742 (14): 00002-7. doi: 10.1016/j.mrrev.2014.01.001.
12. Halazonetis TD, Gorgoulis VG, Bartek J. An oncogene-induced DNA damage model for cancer development. Science 2008; 319 (5868): 1352–1355. doi: 10.1126/science.1140735.
13. Tubio JM, Estivill X. Cancer: when catastrophe strikes a cell. Nature 2011; 470 (7335): 476–477. doi: 10.1038/470476a.
14. Bassaganyas L, Beà S, Escaramís G et al. Sporadic and reversible chromothripsis in chronic lymphocytic leukemia revealed by longitudinal genomic analysis. Leukemia 2013; 27 (12): 2376–2379. doi: 10.1038/leu.2013.127.
15. Federer-Gsponer JR, Quintavalle C, Müller DC et al. Delineation of human prostate cancer evolution identifies chromothripsis as a polyclonal event and FKBP4 as a potential driver of castration resistence. J Pathol 2018; 245 (1): 74–84. doi: 10.1002/path.5052.
16. Jones MJ, Jallepalli PV. Chromothripsis: chromosomes in crisis. Dev Cell 2012; 23 (5): 908–917. doi: 10.1016/j.devcel.2012.10.010.
17. Cortés-Ciriano I, Lee JK, Xi R et al. Comprehensive analysis of chromothripsis in 2,658 human cancers using whole-genome sequencing. [online]. Available from: https: //www.biorxiv.org/content/biorxiv/early/2018/05/30/333617.full.pdf.
18. Cohen A, Sato M, Aldape K et al. DNA copy number analysis of Grade II–III and Grade IV gliomas reveals differences in molecular ontogeny including chromothripsis associated with IDH mutation status. Acta Neuropathol Commun 2015; 3: 34. doi: 10.1186/s40478-015-0213-3.
19. Malhotra A, Lindberg M, Faust GG et al. Breakpoint profiling of 64 cancer genomes reveals numerous complex rearrangements spawned by homology-independent mechanisms. Genome Res 2013; 23 (5): 762–776. doi: 10.1101/gr.143677.112.
20. Molenaar JJ, Koster J, Zwijnenburg DA et al. Sequencing of neuroblastoma identifies chromothripsis and defects in neuritogenesis genes. Nature 2012; 483 (7391): 589–593. doi: 10.1038/nature10910.
21. Mueller S, Engleitner T, Maresch R et al. Evolutionary routes and KRAS dosage define pancreatic cancer phenotypes. Nature 2018; 554 (7690): 62–68. doi: 10.1038/nature25459.
22. Zhang CZ, Leibowitz ML, Pellman D. Chromothripsis and beyond: rapid genome evolution from complex chromosomal rearrangements. Genes Dev 2013; 27 (23): 2513–2530. doi: 10.1101/gad.229559.113.
23. Rücker FG, Dolnik A, Blätte TJ et al. Chromothripsis is linked to TP53 alteration, cell cycle impairment, and dismal outcome in acute myeloid leukemia with complex karyotype. Hematologica 2018; 103 (1): e17–e20. doi: 10.3324/haematol.2017.180497.
24. Magrangeas F, Avet-Loiseau H, Munshi NC et al. Chromothripsis identifies a rare and aggressive entity among newly diagnosed multiple myeloma patients. Blood 2011; 118 (3): 675–678. doi: 10.1182/blood-2011-03-344069.
25. Forment JV, Kaidi A, Jackson SP. Chromothripsis and cancer: causes and consequences of chromosome shattering. Nat Rev Cancer 2012; 12 (10): 663–670. doi: 10.1038/nrc3352.
26. Przybytkowski E, Lenkiewicz E, Barrett MT et al. Chromosome-breakage genomic instability and chromothripsis in breast cancer. BMC Genomics 2014; 15: 579. doi: 10.1186/1471-2164-15-579.
27. Lee KJ, Lee KH, Yoon KA et al. Chromothripsis in treatment resistance in multiple myeloma. Genomics Inform 2017; 15 (3): 87–97. doi: 10.5808/GI.2017.15.3.87.
28. Furgason JM, Koncar RF, Michelhaugh SK et al. Whole genome sequence analysis links chromothripsis to EGFR, MDM2, MDM4, and CDK4 amplification in glioblastoma. Oncoscience 2015; 2 (7): 618–628.
29. Mansfield AS, Peikert T, Smadbeck JB et al. Neoantigenic potential of complex chromosomal rearrangements in mesothelioma. J Thorac Oncol 2019; 14 (2): 276–287. doi: 10.1016/j.jtho.2018.10.001.
30. Nijhawan D, Zack TI, Ren Y et al. Cancer vulnerabilities unveiled by genomic loss. Cell 2012; 150 (4): 842–854. doi: 10.1016/j.cell.2012.07.023.
31. Singh ZN, Richards S, El Chaer F et al. Cryptic ETV6-PDGFRB fusion in a highly complex rearrangement of chromosomes 1, 5 and 12 due to a chromothripsis-like event in a myelodysplastic syndrome/myeloproliferative neoplasm. Leuk Lymphoma 2018; 11: 1–4. doi: 10.1080/10428194.2018.1480774.
32. Bao S, Wu Q, McLendon RE et al. Glioma stem cells promote radioresistance by preferential activation of the DNA damage response. Nature 2006; 444 (7120): 756–760.
33. McDermott DH, Gao JL, Liu Q et al. Chromothriptic cure of WHIM syndrome. Cell 2015; 160 (4): 686–699. doi: 10.1016/j.cell.2015.01.014.
34. Li Y, Schwab C, Ryan S et al. Constitutional and somatic rearrangement of chromosome 21 in acute lymphoblastic leukaemia. Nature 2014; 508 (7494): 98–102. doi: 10.1038/nature13115.
35. Zhang J, Ding L, Holmfeldt L et al. The genetic basis of early T-cell precursor acute lymphoblastic leukaemia. Nature 2012; 481 (7380): 157–163. doi: 10.1038/ nature10725.
36. Fontana MC, Marconi G, Feenstra JD et al. Chromothripsis in acute myeloid leukemia: biological features and impact on survival. Leukemia 2018; 32 (7): 1609–1620. doi: 10.1038/s41375-018-0035-y.
37. Puente XS, Beà S, Valdés-Mas R et al. Non-coding recurrent mutations in chronic lymphocytic leukaemia. Nature 2015; 526 (7574): 519–524. doi: 10.1038/nature14666.
38. Stevens-Kroef M, Weghuis DO, Croockewit S et al. High detection rate of clinically relevant genomic abnormalities in plasma cells enriched from patients with multiple myeloma. Genes Chromosomes Cancer 2012; 51 (11): 997–1006. doi: 10.1002/gcc.21982.
39. Zemanova Z, Michalova K, Buryova H et al. Involvement of deleted chromosome 5 in complex chromosomal aberrations in newly diagnosed myelodysplastic syndromes (MDS) is correlated with extremely adverse prognosis. Leuk Res 2014; 38 (5): 537–544. doi: 10.1016/j.leukres.2014.01.012.
40. Le LP, Nielsen GP, Rosenberg AE et al. Recurrent chromosomal copy number alterations in sporadic chordomas. PLoS One 2011; 6 (5): e18846. doi: 10.1371/journal.pone.0018846.
41. Flynn A, Benn D, Clifton-Bligh R et al. The genomic landscape of phaeochromocytoma. J Pathol 2015; 236 (1): 78–89. doi: 10.1002/path.4503.
42. Mehine M, Kaasinen E, Mäkinen N et al. Characterization of uterine leiomyomas by whole-genome sequencing. N Engl J Med 2013; 369 (1): 43–53.
43. Holzmann C, Markowski DN, Koczan D et al. Cytogenetically normal uterine leiomyomas without MED12-mutations – a source to identify unknown mechanisms of the development of uterine smooth muscle tumors. Mol Cytogenet 2014; 7 (1): 88. doi: 10.1186/s13039-014-0088-1.
44. Cai H, Kumar N, Bagheri HC et al. Chromothripsis-like patterns are recurring but heterogeneously distributed features in a survey of 22,347 cancer genome screens. BMC Genomics 2014; 15: 82. doi: 10.1186/1471-2164-15-82.
45. Parker M, Mohankumar KM, Punchihewa C et al. C11orf95-RELA fusions drive oncogenic NF-κB signalling in ependymoma. Nature 2014; 506 (7489): 451–455. doi: 10.1038/nature13109.
46. Lhotská H, Zemanová Z, Kramář F et al. Molekulárně cytogenetická analýza chromozomových aberací v buňkách nízkostupňových gliomů a její přínos pro klasifikaci nádoru. Klin Onkol 2014; 27 (3): 183–191.
47. Northcott PA, Shih DJ, Peacock J et al. Subgroup-specific structural variation across 1,000 medulloblastoma genomes. Nature 2012; 488 (7409): 49–56. doi: 10.1038/nature11327.
48. Brastianos PK, Horowitz PM, Santagata S et al. Genomic sequencing of meningiomas identifies oncogenic SMO and AKT1 mutations. Nat Genet 2013; 45 (3): 285–289. doi: 10.1038/ng.2526.
49. Ambros IM, Brunner C, Abbasi R et al. Ultra-high density SNParray in neuroblastoma molecular diagnostics. Front Oncol 2014; 4: 202. doi: 10.3389/fonc.2014. 00202.
50. McEvoy J, Nagahawatte P, Finkelstein D et al. RB1 gene inactivation by chromothripsis in human retinoblastoma. Oncotarget 2014; 5 (2): 438–450. doi: 10.18632/oncotarget.1686.
51. Nones K, Waddell N, Wayte N et al. Genomic catastrophes frequently arise in esophageal adenocarcinoma and drive tumorigenesis. Nat Commun 2014; 5: 5224. doi: 10.1038/ncomms6224.
52. Bass AJ, Lawrence MS, Brace LE et al. Genomic sequencing of colorectal adenocarcinomas identifies a recurrent VTI1A-TCF7L2 fusion. Nat Genet 2011; 43 (10): 964–968. doi: 10.1038/ng.936.
53. Kloosterman WP, Hoogstraat M, Paling O et al. Chromothripsis is a common mechanism driving genomic rearrangements in primary and metastatic colorectal cancer. Genome Biol 2011; 12 (10): R103. doi: 10.1186/gb-2011-12-10-r103.
54. Fernandez-Banet J, Lee NP, Chan KT et al. Decoding complex patterns of genomic rearrangement in hepatocellular carcinoma. Genomics 2014; 103 (2–3): 189–203. doi: 10.1016/j.ygeno.2014.01.003.
55. Jiang Z, Jhunjhunwala S, Liu J et al. The effects of hepatitis B virus integration into the genomes of hepatocellular carcinoma patients. Genome Res 2012; 22 (4): 593–601. doi: 10.1101/gr.133926.111.
56. Fraser M, Sabelnykova VY, Yamaguchiet TN et al. Genomic hallmarks of localized, non-indolent prostate cancer. Nature 2017; 541 (7637): 359–364. doi: 10.1038/nature20788.
57. Lapuk AV, Wu C, Wyatt AW et al. From sequence to molecular pathology, and a mechanism driving the neuroendocrine phenotype in prostate cancer. J Pathol 2012; 227 (3): 286–297. doi: 10.1002/path.4047.
58. Nik-Zainal S, Van Loo P, Wedge DC et al. The life history of 21 breast cancers. Cell 2012; 149 (5): 994–1007. doi: 10.1016/j.cell.2012.04.023.
59. Morrison CD, Liu P, Woloszynska-Read A et al. Whole-genome sequencing identifies genomic heterogeneity at a nucleotide and chromosomal level in bladder cancer. Proc Natl Acad Sci U.S.A. 2014; 111 (6): E672–E681. doi: 10.1073/pnas.1313580111.
60. Govindan R, Ding L, Griffith M et al. Genomic landscape of non-small cell lung cancer in smokers and never-smokers. Cell 2012; 150 (6): 1121–1134. doi: 10.1016/j.cell.2012.08.024.
61. Poaty H, Coullin P, Peko JF et al. Genome-wide high-resolution aCGH analysis of gestational choriocarcinomas. PLoS One 2012; 7 (1): e29426. doi: 10.1371/journal.pone.0029426.
62. Hirsch D, Kemmerling R, Davis S et al. Chromothripsis and focal copy number alterations determine poor outcome in malignant melanoma. Cancer Res 2013; 73 (5): 1454–1460. doi: 10.1158/0008-5472.CAN-12-0928.
63. Akbani R, Akdemir KC, Aksoy BA et al. Genomic classification of cutaneous melanoma. Cell 2015; 161 (7): 1681–1696. doi: 10.1016/j.cell.2015.05.044.
64. van Engen-van Grunsven AC, Baar MP, Pfundt R et al. Whole-genome copy-number analysis identifies new leads for chromosomal aberrations involved in the oncogenesis and metastastic behavior of uveal melanomas. Melanoma Res 2015; 25 (3): 200–209. doi: 10.1097/CMR.0000000000000152.
Labels
Paediatric clinical oncology Surgery Clinical oncologyArticle was published in
Clinical Oncology
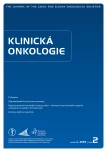
2019 Issue 2
Most read in this issue
- Chromothripsis – Extensive Chromosomal Rearrangements and Their Significance in Cancer
- Gorlin-Goltz syndrome
- Oligometastatic Prostate Cancer
- Hepatic Injury Induced by a Single Dose of Nivolumab – a Case Report and Literature Review