Antiatherosclerotic and antiinflammatory activities of SGLT2 inhibitors
Authors:
Ján Murín
Authors‘ workplace:
I. interná klinika LFUK a UNB, Nemocnica Staré Mesto, Bratislava
Published in:
AtheroRev 2021; 6(1): 25-29
Category:
Overview
Type 2 diabetes mellitus (T2DM) is a systemic disease with many cardiovascular and renal complications. Treatment of DM should be today with new antidiabetic drugs as SGLT2 inhibitors and GLP-1 RA, as they can reduce mentioned complications. In this article we concentrate on SGLT2 inhibitors and their activities: antiatherosclerotic (blood pressure reduction, reduction of epicardial and visceral fat mass, inhibition of lipogenesis, autophagy activation, support for angiogenesis, mTOR inhibition, mitochondrial function improvements, free oxygen radicals reduction) and antiinflammatory (betahydroxybutyrate production increase which has antiinflammatory and antiremodeling activities, free oxygen radicals reduction, reduction of uricaemia, anti-IL-1β activity, reduction of activation of NLRP3 inflammasome, serum levels reduction of TNF-receptor 1 but also IL-6 and matrix metalloproteinase 7). These mechanisms are thoroughly investigated in last years and they can explain CV and renal benefits of SGLT2 inhibitors in diabetic patients.
Keywords:
antiatherosclerotic activities – antiinflammatory activities – diabetes mellitus – SGLT2 inhibitors – antiinflammatory activities – diabetes mellitus – SGLT2 inhibitors
Sources
-
Cho NH, Shaw JE, Karuranga S et al. IDF Diabetes atlas: global estimates of diabetes prevalence for 2017 and projections for 2045. Diabetes Res Clin Pract 2018; 138: 271–281. Dostupné z DOI: <http://10.1016/j.diabres.2018.02.023>.
-
Benjamin EJ, Muntner P, Alonso A et al. Heart disease and stroke statistics-2019 update: a report from the American Heart Association. Circulation 2019; 139(10): e506–528. Dostupné z DOI: <http://dx.doi.org/10.1161/CIR.0000000000000659>.
-
Zelniker TA, Wiviott SD, Raz I et al. SGLT2 inhibitors for primary and secondary prevention of cardiovascular and renal outcomes in type 2 diabetes: a systematic review and meta-analysis of cardiovascular outcome trials. Lancet 2019; 393(10166): 31–39. Dostupné z DOI: <http://dx.doi.org/10.1016/S0140–6736(18)32590-X>.
-
Kristsensen SL, Rorth R, Jhund PS et al. Cardiovascular, mortality, and kidney outcomes with GLP-1 receptor agonists in patients with type 2 diabetes: a systematic review and meta-analysis of cardiovascular outcome trials. Lancet Diabetes Endocrinol 2019; 7(10): 776–785. Dostupné z DOI: <http://dx.doi.org/10.1016/S2213–8587(19)30249–9>.
-
McMurray JJ, Solomon SD, Inzuccchi SE et al. Dapagliflozin in patients with heart failure and reduced ejection fraction. N Engl J Med 2019; 381(21): 1995–2008. Dostupné z DOI: <http://dx.doi.org/10.1056/NEJMoa1911303>.
-
Packer M, Anker SD, Butler J, et al. Cardiovascular and renal outcomes with empagliflozin in heart failure. N Engl J Med 2020; 383(15): 1413–1424. Dostupné z DOI: <http://dx.doi.org/10.1056/NEJMoa2022190>.
-
Heerspink HJL, Stefansson BV, Correa-Rotter R et al. Dapagliflozín in patients with chronic kidney disease. N Engl J Med 2020; 383(15): 1436–1446. Dostupné z DOI: <http://dx.doi.org/10.1056/NEJMoa2024816>.
-
Mazidi M, Rezaie P, Gao HK et al. Effect of sodium-glucose cotransport-2 inhibitors on blood pressure in people with type 2 diabetes mellitus: a systematic review and meta-analysis of 43 r andomized control trials with 22 528 patients. J Am Heart Assoc 2017; 6(6): e004007. Dostupné z DOI: <http://dx.doi.org/10.1161/JAHA.116.004007>.
-
Weber MA, Mansfield TA, Cain VA et al. Blood pressure and glycaemic effects of dapagliflozin versus placebo in patients with type 2 diabetes on combination antihypertensive therapy: a randomised, double-blind, placebo-controlled, phase 3 study. Lancet Diabetes Endocrinol 2016; 4(3): 211–220. Dostupné z DOI: <http://dx.doi.org/10.1016/S2213–8587(15)00417–9>.
-
Kario K, Bohm M, Mahfoud F et al. Twenty-four-hour ambulatory blood pressure reduction patterns after renal denervation in the SPYRAL HTN-OFF MED trial. Circulation 2018; 138(15): 1602–1604. Dostupné z DOI: <http://dx.doi.org/10.1161/CIRCULATIONAHA.118.035588>.
-
Raggi P, Gadiyaram V, Zhang C et al. Statins reduce epicardial adipose tissue attenuation independent of lipid lowering: a potential pleiotropic effect. J Am Heart Assoc 2019; 8(12): e013104. Dostupné z DOI: <http://dx.doi.org/10.1161/JAHA.119.013104>.
-
Sato T, Aizawa Y, Yuasa S et al. The effect of dapagliflozin treatment on epicardial adipose tissue volume. Cardiovasc Diabetol 2018; 17(1): 6. Dostupné z DOI: <http://dx.doi.org/10.1186/s12933–017–0658–8>.
-
Iborra-Egea O, Santiago-Vacas E, Yurista SR et al. Unraveling the molecular mechanism of action of empagliflozin in heart failure with reduced ejection fraction with or without diabetes. JACC Basic Transl Sci 2019; 4(7): 831–840. Dostupné z DOI: <http://dx.doi.org/10.1016/j.jacbts.2019.07.010>.
-
Miyachi Y, Tsuchiya K, Shiba K et al. A reduced M1-like/M2-like ratio of macrophages in healthy adipose tissue expansion during SGLT2 inhibition. Sci Rep 2018; 8(1): 16113. Dostupné z DOI: <http://dx.doi.org/10.1038/s41598–018–34305-x>.
-
Osataphan S, Macchi C, Singhal G et al. SGLT2 inhibition reprograms systemic metabolism via FGF21-dependent and – independent mechanisms. JCI Insight 2019; 4(5): e123130. Dostupné z DOI: <http://dx.doi.org/10.1172/jci.insight.123130>.
-
Winder WW, Hardie DG. AMP-activated protein kinase, a metabolic master switch: possible roles in type 2 diabetes. Am J Physiol 1999; 277(1): E1-E10. Dostupné z DOI: <http://dx.doi.org/10.1152/ajpendo.1999.277.1.E1>.
-
Jeon SM. Regulation and function of AMPK in physiology and disease. Exp Mol Med 2016; 48(7): e245. Dostupné z DOI: <http://dx.doi.org/10.1038/emm.2016.81>.
-
Ouchi N, Shibata R, Walsh K. AMP-activated protein kinase signaling stimulates VEGF expression and angiogenesis in skeleted muscle. Circul Res 2005; 96(8): 838–836. Dostupné z DOI: <http://dx.doi.org/10.1161/01.RES.0000163633.10240.3b>.
-
Mukherjee S, Mukherjee U. A comprehensive review of immunosuppression used for liver transplantation. J Transplant 2009: 701464. Dostupné z DOI: <http://dx.doi.org/10.1155/2009/701464>.
-
Lipton JO, Sahin M, The neurology of mTOR. Neuron 2014; 84(2): 275–291. Dostupné z DOI: <http://dx.dpoi.org/10.1016/j.neuron.2014.09.034>.
-
Hay N, Sonenberg N. Upstream and downstream of mTOR. Genes Dev 2004; 18(16): 1926–1945. Dostupné z DOI: <http://dx.doi.org/10.1101/gad.1212704>.
-
Yin Y, Hua H, Li M et al. mTORC2 promotes type I IGF receptor and insulin receptor activation through the tyrosine kinase activity of mTOR. Cell Res 2016; 26(1): 46–65. Dostupné z DOI: <http://dx.doi.org/10.1038/cr.2015.133>.
-
Tokunaga C, Yoshino K, Yonezawa K. mTOR integrates amino acid- and energy-sensing pathways. Biochem Biophys Res Commun 2004; 313(2): 443–446. Dostupné z DOI: <http://dx.doi.org/10.1016/j.bbrc.2003.07.019>.
-
Wipperman MF, Montrose DC, Gotto AM et al. Mammalian Target of Rapamycin – a metabolic rheostat for regulating adipose tissue function and cardiovascular health. Am J Pathol 2019; 189(3): 492–501. Dostupné z DOI: <http://dx.doi.org/10.1016/j.ajpath.2018.11.013>.
-
Beevers CS, Li F, Liu L et al. Curcumin inhibits the mammalian TOR-mediated signaling pathways in cancer cells. Int J Cancer 2006; 119(4): 757–764. Dostupné z DOI: <http://dx.doi.org/10.1002/ijc.21932>.
-
Huang S, Houghton PJ. Mechanisms of resistance to rapamycins. Drug Resistance Updates 2001; 4(6): 378–391. Dostupné z DOI: <http://dx.doi.org/10.1054/drup.2002.0227>.
-
Powers RW, Kaeberlein M, Caldwell SD et al. Extension of chronological life span in yeast by decreased TOR pathway signaling. Genes Dev 2006; 20(2): 174–184. Dostupné z DOI: <http://dx.doi.org/10.1101/gad.1381406>.
-
Brioche T, Pagano AF, Py G et al. Muscle wasting and aging: experimental models, fatty infiltrations and prevention. Mol Aspects Med 2016; 50: 56–87. Dostupné z DOI: <http://dx.doi.org/10.1016/j.mam.2016.04.006>.
-
Xu, K. Liu P, Wei W. mTOR signaling in tumorigenesis. Biochim Biophys Acta 2014; 1846(2): 638–654. Dostupné z DOI: <http://dx.doi.org/10.1016/j.bbcan.2014.10.007>.
-
Zoncu R, Efeyan A, Sabatini DM. mTOR: form growth signal integration to cancer, diabetes, and ageing. Nat Rev Mol Cell Biol 2011; 12(1): 21–35. Dostupné z DOI: <http://dx.doi.org/10.1038/nrm3025>.
-
Nishimura T, Nakatake Y, Konishi M et al. Identification of a novel FGF, FGF21, preferentially expressed in the liver. Biochim Biophys Acta 2000; 1492(1): 203–206. Dostupné z DOI: <http://dx.doi.org/10.1016/s0167–4781(00)00067–1>.
-
Talukdar S, Owen BM, Song P et al. FGF21 regulates sweet and alcohol preference. Cell metab 2016; 23(2): 344–349. Dostupné z DOI: <http://dx.doi.org/10.1016/j.cmet.2015.12.008>.
-
Markan KR, Naber MC, Ameka MK et al. Circulating FGF21 is liver derived and enhances glucose uptake during refeeding and overfeeding. Diabetes 2014; 63(12): 4057–4063. Dostupné z DOI: <http://dx.doi.org/10.2337/db14–0595>.
-
Kharitonenkov A, Shiyanova TL, Koester A et al. FGF21 as a novel metabolic regulator. J Clin Investig 2005; 115(6): 1627–1635. Dostupné z DOI: <http://dx.doi.org/10.1172/JCI23606>.
-
Cheng X, Zhu B, Jiang F et al. Serum FGF-21 levels in type 2 diabetic patients. Endocr Res 2011; 36(4): 142–148. Dostupné z DOI: <http://dx.doi.org/10.3109/07435800.2011.558550>.
-
Kenny HC, Abel ED. Heart failure in type 2 diabetes mellitus. Circ Res 2019; 124(1): 121–141. Dostupné z DOI: <http://dx.doi.oeg/10.1161/CIRCRESAHA.118.311371>.
-
Jia G, Hill MA, Sowers JR. Diabetic cardiomyopathy: An update of mechanism contributing to this clinical entity. Circ Res 2018; 122(4): 624–638. Dostupné z DOI: <http://dx.doi.org/10.1161/CIRCRESAHA.117.311586>.
-
Zelniker TA, Braunwald E. Clinical benefit of cardiorenal effects of sodium-glucose cotransporter 2 inhibitors. JACC state-of-the-art review. J Am Coll Cardiol 2020; 75(4): 436–447. Dostupné z DOI: <http://dx.doi.org/10.1016/j.jacc.2019.11.036>.
-
Uthman L, Homayr A, Juni RP et al. Empagliflozin and dapagliflozin reduce ROS generation and restore NO biovailability in tumor necrosis factor alfa – stimulated human coronary arterial endothelial cells. Cell Physiol Biochem 2019; 53(5): 865–886. Dostupné z DOI: <http://dx.doi.org/10.33594/000000178>.
-
Endemann DH, Schiffrin EL. Endothelial dysfunction. J Am Soc Nephrol 2004; 15(8):1983–1992. Dostupné z DOI: <http://dx.doi.org/10.1097/01.ASN.0000132474.50966.DA>.
-
Gaspari T, Spizzo I, Liu H et al. Dapagliflozin attenuates human vascular endothelial cell activation and induces vasorelaxation: a potential mechanism for inhibition of atherogenesis. Diabetes Vasc Dis Res 2017; 15(1): 64–73. Dostupné z DOI: <http://dx.doi.org/10.1177/1479164117733626>.
-
Juni RP, Kuster DWD, Goebel M et al. Cardiac microvascular endothelial enhancement of cardiomyocyte function is impaired by inflammation and restored by empagliflozin. JACC Basic Transl Sci 2019; 4(5): 575–591. Dostupné z DOI: <http://dx.doi.org/10.1016/j.jacbts.2019.04.003>.
-
Li H, Shin SE, Seo MS et al. The anti-diabetic drug dapagliflozin induces vasodilation via activation of PKG and Kv chennels. Life Sci 2018; 197: 46–55. Dostupné z DOI: <http://dx.doi.org/10.1016/j.lfs.2018.01.032>.
-
Tran DH, Wang ZV. Glucose metabolism in cardiac hypertrophy and heart failure. J Am Heart Assoc 2019; 8(12): e012673. Dostupné z DOI: <http://dx.doi.org/10.1161/JAHA.119.012673>.
-
Sowton AP, Griffin JL, Murray AJ. Metabolic profiling of the diabetic heart: Toward a richer picture. Front Physiol 2019; 10: 639. Dostupné z DOI: <http://dx.doi.org/10.3389/fphys.2019.00639>.
-
Ferrannini E, Baldi S, Frascerra S et al. Shift to fatty substrate utilization in response to sodium-glucose cotransporter 2 inhibition in subjects without diabetes and patients with type 2 diabetes. Diabetes 2016; 65(5): 1190–1195. Dostupné z DOI: <http://dx.doi.org/10.2337/db15–1356>.
-
Horton JL, Davidson MT, Kurishima C et al. The failling heart utilizes 3-hydroxybutyrate as a metabolic stress defense. JCI Insight 2019; 4(4): e124079. Dostupné z DOI: <http://dx.doi.org/10.1172/jci.insight.124079>.
-
Youm YH, Nguyen KY, Grant RW et al. The ketone metabolite beta-hydroxybutyrate blocks NLRP3 inflammasome-mediated inflammatory disease. Nat Med 2015; 21(3): 263–269. Dostupné z DOI: <http://dx.doi.org/10.1038/nm.3804>.
-
Bonnet F, Sheen AJ. Effects of SGLT2 inhibitors on systemic and tissue low-grade inflammation: The potential contribution to diabetes complications and cardiovascular disease. Diabetes Metab 2018; 44(6): 457–464. Dostupné z DOI: <http://dx.doi.org/10.1016/j.diabet.2018.09.005>.
-
Marwick TH, Ritchie R, Shaw JE et al. Implications of underlying mechanisms for the recognition and management of diabetic cardiomyopathy. J Am Coll Cardiol 2018; 71(3): 339–351. Dostupné z DOI: <http://dx.doi.org/10.1016/j.jacc.2017.11.019>.
-
Zelniker TA, Jarolim P, Scirica BM et al. Biomarker of collagen turnover (C-terminal telopeptide) and prognosis in patients with non- ST –elevation acute coronary syndromes. J Am Heart Assoc 2019; 8(9): e011444. Dostupné z DOI: <http://dx.doi.org/10.1161/JAHA.118.011444>.
-
Ye Y, Bajaj M, Yang HC et al. SGLT-2 inhibition with dapagliflozin reduces the activation of the NLRP3/ASC inflammasome and attenuates the development of diabetic cardiomyopathy in mice with type 2 diabetes. Further augmentation of the effects with saxagliptin, a DPP4 inhibitor. Cardiovasc Drugs Ther 2017; 31(2): 119–132. Dostupné z DOI: <http://dx.doi.org/10.1007/s10557–017–6725–2>.
-
Brenner D. Regulation of TNF signaling: live or let die. Nat Rev Immunol 2015; 15(6):362–374. Dostupné z DOI: <http://dx.doi.org/10.1038/nri3834>.
-
Vandenabeele P. Molecular mechanisms of necroptosis: an ordered cellular explosion. Nat Rev Moll Cell Biol 2010; 11(10):700–714. Dostupné z DOI: <http://dx.doi.org/10.1038/nrm2970>.
-
Fischer R, Maier O. Interrelation of oxidative stress and inflammation in neurodegenerative disease: role of TNF. Oxid Med Cell Longev 2015; 2015: 610813. Dostupné z DOI: <http://dx.doi.org/10.1155/2015/610813>.
-
Han D. Redox regulation of TNF signaling. Antioxid Redox Signal 2009; 11(9): 2245–2263. Dostupné z DOI: <http://dx.doi.org/10.1089/ars.2009.2611>.
-
El-Daly M, Pulakazhi M, Venu VK, et al. Hyperglycaemic impairment of PAR2-mediated vasodilation: prevention by inhibition of aortic endothelial sodium-glucose-co-transporter-2 and minimizing oxidative stress. Vascul Pharmacol 2018; 109:56–71. Dostupné z DOI: <http://dx.doi.org/10.1016/j.vph.2018.06.006>.
-
Mancini SJ, Boyd D, Katwan OJ et al. Canagliflozin inhibits interleukin-1beta-stimulated cytokine and chemokine secretion in vascular endothelial cells by AMP-activated protein kinase-dependent and –independent mechanisms. Sci Rep 2018; 8(1): 5276. Dostupné z DOI: <http://dx.doi.org/10.1038/s41598–018–23420–4>.
-
Novikov A, Fu Y, Huang W et al. SGLT2 inhibition and renal urate excretion: role of luminal glucose, GLUT9, and URAT1. Am J Physiol Renal Physiol 2019; 316(1): F173-F185. Dostupné z DOI: <http://dx.doi.org/10.1152/ajprenal.00462.2018>.
-
Wilcox CS, Shen W, Boulton DW et al. Interaction between the sodium-glucose-linked transporter 2 inhibitor dapagliflozin and the loop diuretic bumetanide in normal human subjects. J Am Heart Assoc 2018; 7(4): e007046. Dostupné z DOI: <http://dx.doi.org/10.1161/JAHA.117.007046>.
-
Lytvyn Y, Perkins BA, Cherney DZ. Uric acid as a biomarker and a therapeutic target in diabetes. Can J Diabetes 2015; 39(3): 239–246. Dostupné z DOI: <http://dx.doi.org/10.1016/j.jcjd.2014.10.013>.
-
Alicic RZ, Rooney MT, Tuttle KR. Diabetic kidney disease: Challenges, progress, and possibilities. Clin J Am Soc Nephrol 2017; 12(12): 2032–2045. Dostupné z DOI: <http://dx.doi.org/10.2215/CJN.11491116>.
-
Woods TC, Satou R, Miyata K et al. Canagliflozin prevents intrarenal angiotensinogen augmentation and mitigates kidney injury and hypertension in mouse model of type 2 diabetes mellitus. Am J Nephrol 2019; 49(4): 331–342. Dostupné z DOI: <http://dx.doi.org/10.1159/000499597>.
-
Yaribeygi H, Atkin SL, Butler AE et al. Sodium-glucose cotransporter inhibitors and oxidative stress: an update. J Cell Physiol 2019; 234(4): 3231–3237. Dostupné z DOI: <http://dx.doi.org/10.1002/jcp.26760>.
-
Birnbaum Y, Bajaj M, Yang HC et al. Combined SGLT2 and DPP4 inhibition reduces the activation of the NLRP3/-ASC inflammasome and attenuates the development of diabetic nephropathy in mice with type 2 diabetes. Cardiovasc Drugs Ther 2018; 32(2): 135–145. Dostupné z DOI: <http://dx.doi.org/10.1007/s10557–018–6778-x>.
-
Masters SL, Dunne A, Subramanian SL et al. Activation of the NLRP3 inflammasome by islet amyloid polypeptide provides a mechanism for enhanced IL-1beta in type 2 diabetes. Nat Immunol 2010; 11(10): 897–904. Dostupné z DOI: <http://dx.doi.org/10.1038/ni.1935>.
-
Heerspink HJL, Perco P, Mulder S et al. Canagliflozin reduces inflammation and fibrosis biomarkers: a potential mechanism of action for beneficial effects of SGLT2 inhibitors in diabetic kidney disease. Diabetologia 2019; 62(7): 1154–1166. Dostupné z DOI: <http://dx.doi.org/10.1007/s00125–019–4859–4>.
Labels
Angiology Diabetology Internal medicine Cardiology General practitioner for adultsArticle was published in
Athero Review
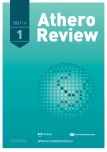
2021 Issue 1
Most read in this issue
- Inclisiran: the first siRNA approved by European Medicines Agency for treatment of dyslipidemias
- Second consensus statement of European Atherosclerosis Society on low-density lipoproteins: statement of Czech Society for Atherosclerosis
- Low concentration of plasma cholesterol and type 2. diabetes mellitus
- Early cardiovascular risk intervention means cummulation of positive impacts in time